Expression systems, among which the recombinant vector pET, are widely used in biotechnology to introduce foreign genes into prokaryotes or simple eukaryotes. This technique makes it possible to eventually obtain recombinant proteins from those organisms that should not produce these proteins in the natural environment. For example, one of the most widespread bacteria in which genes of interest are actively placed is E. coli. The use of E. coli for genetic engineering purposes is due to the widespread occurrence of the prokaryote, its genomic simplicity, its complete study, and hence its relatively cheap production. For example, insulin preparation for diabetic patients has already traditionally been synthesized precisely from E. coli, into which the genes of a healthy human encoding the synthesis of this protein are placed (Zieliński et al., 2019). The use of a subtype of E. coli, namely Escherichia coli BL21(DE3), is widespread: this commercial bacterium contains the DE3 prophage, which was previously isolated from bacteriophage λ. At the same time, it is worth mentioning that as recombinant vectors, especially in conjunction with E. coli, the pET system is just as widely used. pET is not a specific plasmid but rather a group of recombinant vectors that can be modified for individual cases. For example, the pET-28a(+) vector is very popular, with a weight of up to 5.4 kb, as well as integrated T7 promoter genes. Figure 1 below shows the large variety of pET systems for incorporation into bacteria, which means that discussion between students and the supervisor should at least address the motivation for choosing a particular vector.
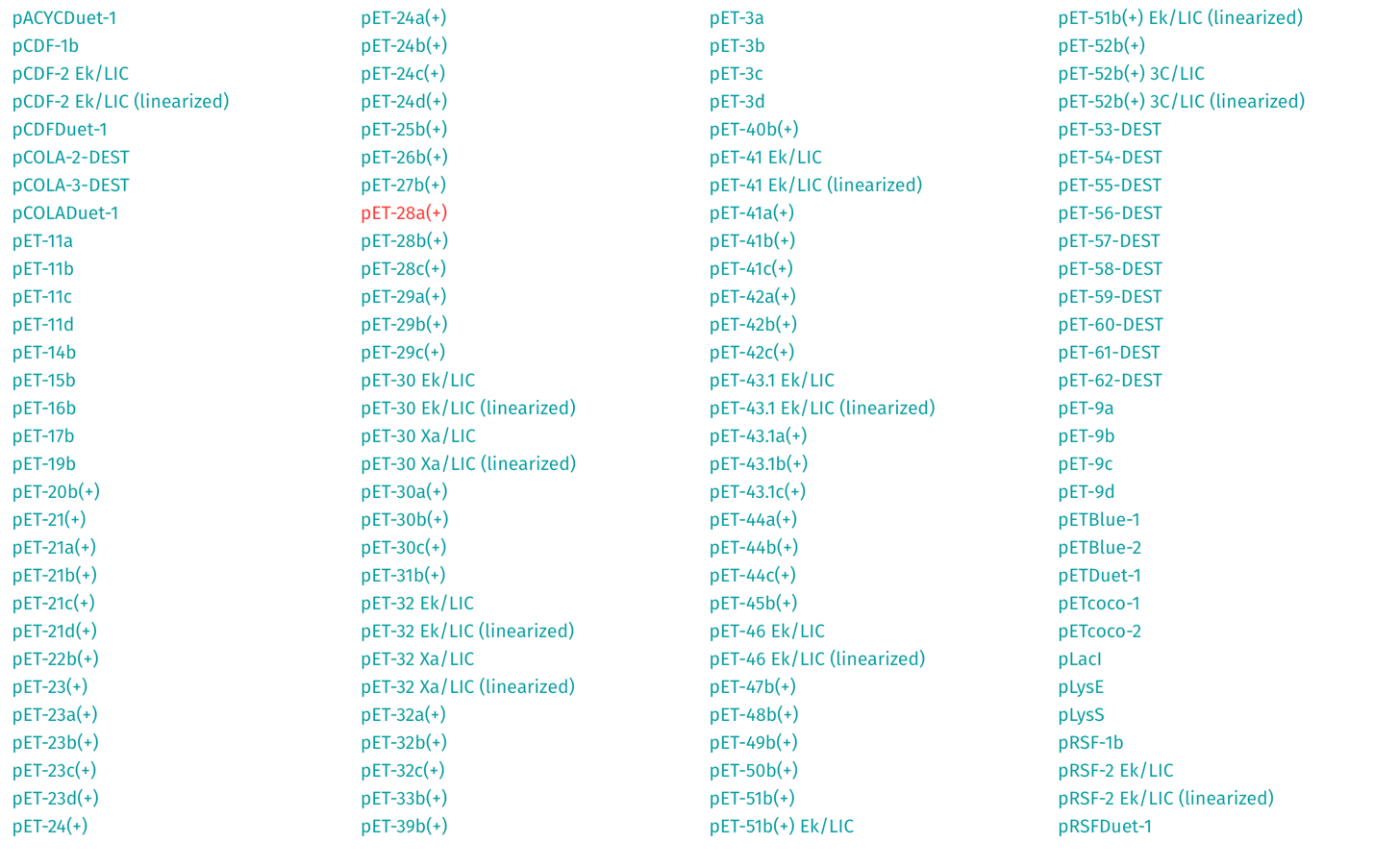
It should be understood that pET vectors are traditionally used in such bacteria that lack the RNA polymerase T7 gene. This is done deliberately because expression of embedded genes of interest in E. coli could be detrimental to the prokaryote, and the presence of RNA polymerase T7 would lead to instability of expression, suppressing it. However, Escherichia coli BL21(DE3), which the supervisor proposed to use, has a built-in gene for this enzyme. Consequently, placing the recombinant pET system inward is associated with serious risks of killing such cells or unstable expression, which means that conditions are created to search for additional transformation strategies. In particular, there are at least two solutions to this problem, one of which is excellent for the student. First, it is possible to place a pET vector into a bacterium that does not contain the T7 RNA polymerase gene, which means that expression, in this case, will be stable. Already then, this prokaryote is infected with a phage carrying the RNA polymerase gene T7: in this way, Escherichia coli BL21, initially combined with pET, can be obtained from Escherichia coli BL21(DE3). In this idea, it is important to say that Escherichia coli BL21(DE3) differs from Escherichia coli BL21 precisely in the presence of the RNA polymerase T7 expression gene. The second option is to use such a strain of Escherichia coli BL21(DE3) that would contain the T7 RNA polymerase gene but under the control of the lacUV5 promoter.
It is worth mentioning that lacUV5 is a mutated promoter located in the lac operon of E. coli: its presence is characteristic of Escherichia coli BL21(DE3). Under natural conditions in this bacterium, a repressor exists in the lac operon that suppresses plasmid gene expression. More specifically, the repressor is thought to bind to the lac operon DNA, which causes a configuration change: the DNA molecule bends 40 degrees, which prevents transcription (Biologics International Corp, 2018). However, indexing this cell with lactose allows gene expression to be triggered because the repressor no longer has physical access to the DNA operon. In other words, the presence of lactose ensures that the lac repressor can no longer bind to the DNA sites on the plasmid, and thus gene expression is restored. This strategy creates additional discussion for the professor and the student since the addition of normal lactose is unlikely to lead to the expected result. Instead, scientists should consider which lactose isomer to use for bacterial culture: it could be, for example, isopropyl β-D-1-thiogalactopyranoside (IPTG), which is chemically allolactose. The use of IPTG, in turn, creates an additional advantage because controlling the concentration of the disaccharide affects the intensity of expression.
Performing laboratory procedures with Escherichia coli BL21(DE3) will be associated with technical complexity, as the student will need to use a complex of methods. Specifically, the student will need to use PCR techniques to amplify the gene of interest and restriction techniques with the addition of DNA ligases to glue the gene and pET plasmid together. In addition, electrophoresis and purification techniques for the resulting cloned genes will be required. Incubation at a specific temperature will be necessary to cultivate competent cells, and IPTG induction (addition) will be useful to trigger gene expression. Protein isolation is made possible by the microcentrifugation of samples.
References
Biologics International Corp. (2018). IPTG induction theory. BIC. Web.
pET-28a(+). (n.d.). SnapGene. Web.
Zieliński, M., Romanik-Chruścielewska, A., Mikiewicz, D., Łukasiewicz, N., Sokołowska, I., Antosik, J.,… & Płucienniczak, A. (2019). Expression and purification of recombinant human insulin from E. coli 20 strain. Protein Expression and Purification, 157, 63-69. Web.