Plate Tectonics and Continental Drift
Within the framework of modern geodynamics, it is proved scientifically and empirically that the surface of the Earth is divided into several plates. The Earth’s exterior looks like a mosaic embracing eight large and six small plates completely covering it.
The boundaries of the lithospheric plates are expressed by active seismicity and magmatism and do not always coincide with the boundaries of the continents. For instance, the plate covering North America (the North American Plate) spreads not only across the North American mainland but also across the western area of the Atlantic Ocean.
Plate tectonics is a geodynamic concept based on the concept of the Earth’s lithosphere as a collection of moving lithospheric plates, which continuously interact with each other in the process of moving. The plate movement is controlled by convection mantle flows; this movement is an element of the heat transfer system from the Earth’s center to its surface. The lower layers of the lithospheric plates are formed by the rocks of the mantle; the upper ones are the crust of either only oceanic type or both oceanic and continental. The oceanic crust has an average thickness of no more than 7 kilometers and consists mainly of basalt and rocks of similar composition. The continental crust is much thicker: from 35 to 70 kilometers. This type of crust consists of a wide range of rocks, among which, however, granites predominate. The interaction of moving plates leads to the formation of zones of seismic and volcanic activity at the boundaries between them.
Tectonic processes began to play a significant role in the paleogeography of the Earth, apparently at about 3.2 billion years. Global reconstructions of tectonic history using a complex of geological and geophysical (paleomagnetic) data obtained from continents and from the ocean floor are limited to the nearest 200 million years. This is the maximum age of the oceanic crust since the older ocean floor is absorbed in the subduction process of one plate under or beneath another one near convergent boundaries.
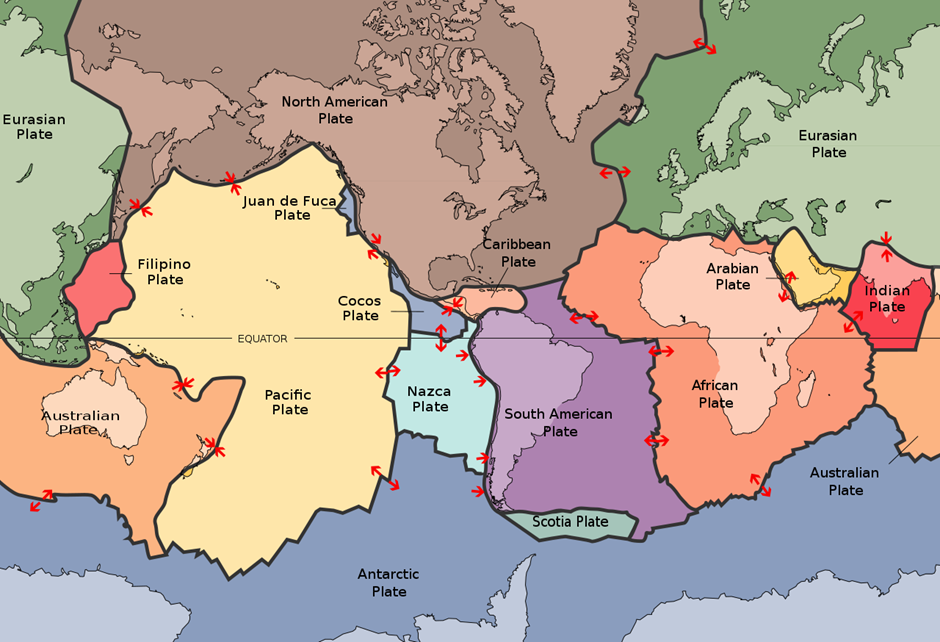
The ratios of the plates are determined by three main types of boundaries – divergent, convergent and transform. Specifically, a divergent boundary is the one that is located between two diverging lithospheric plates. In the ocean, they are expressed by mid-ocean ridges, which, depending on the spreading rate, have a different morphology.
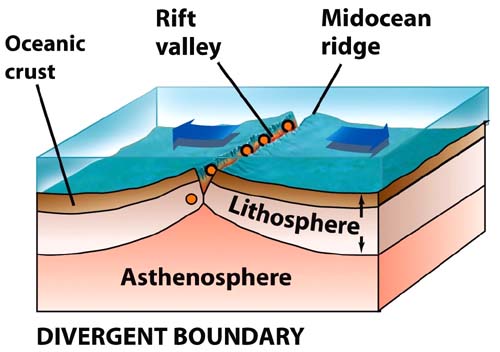
Lately, in the mid-ocean ridge located at the bottom of the Atlantic Ocean, a new oceanic crust is being formed. Within this process, the floor of the Atlantic expands evenly. In other parts of the world the opposite process takes place – for example, in the northwestern Pacific Ocean, the oceanic crust moves under the continent of Eurasia and plunges into the Earth’s mantle. As a result, the total surface area of the Earth does not change, as the expansion of the floor in the Atlantic is balanced out by the contraction of the Pacific Ocean.
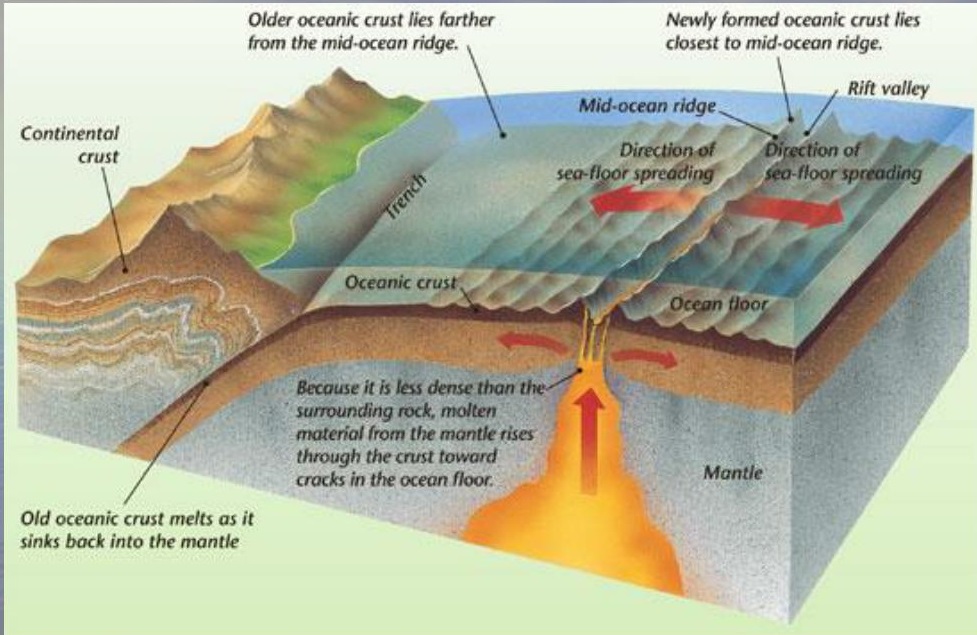
The horizontal shifts of the plates occur because of the mantling of heat-gravity flows (this process is called convection). The mantle consists of silicate rocks rich in iron and magnesium in relation to the overlying crust. High temperatures in the mantle make the silicate material plastic enough to allow convection of material in the mantle, which emerges to the surface through faults in tectonic plates. There is a significant difference between temperature (that equals approximately 5000°C) between the planet’s central areas and the temperature of the Earth’s surface. This very differentiation of temperatures is the source of energy for these gravity flows. Heated structures expand in the significant Earth zones, but the density rate declines. These structures rocket to the surface, making way to the getting down of heavier and colder masses, which have shared part of the heart waves to the Earth’s crust.
Thermal convection in the mantle material acts out as an effective mechanism for the transfer of thermal energy from the Earth’s core. This mechanism is called convective cells, which can grow up to several thousand kilometers in size. Above the ascending flows of mantle matter which are hot and less dense, there are zones of spreading of the ocean floor. In the zones of the subduction process, there are the descending currents of denser and cooled mantle matters spreading along with the lithospheric plates. The plate movement is the result of the dense adhesion transpiring in the upper mantle matter. This movement is carried out convectively on the lithosphere’s uneven base.
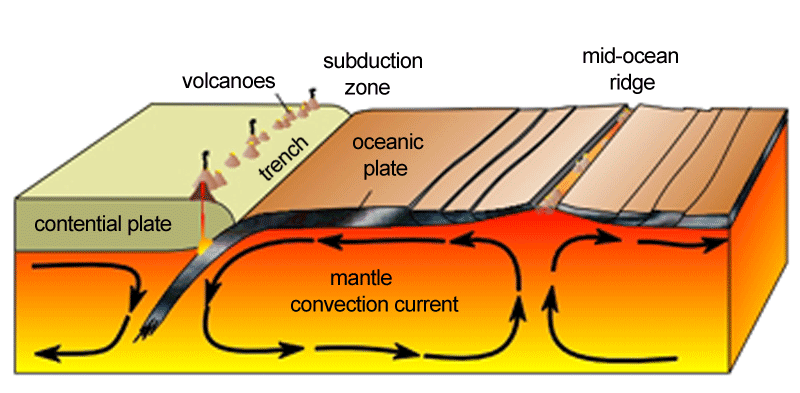
Riftogenesis is the horizontal stretching process occurring in the lithosphere. Oceanic basins are the places where riftogenesis boundaries are attached to mid-ocean ridges and continental rifts. The definition “rift” is used to detect linear and formed structures during the process of the Earth’s crust stretching. Rifts forming either in oceanic or continental crusts can create a complete global system relatively oriented on the geoid axis. It is a case of the continental rift evolution entailing the continuity rupture of the continental crust. This phenomenon might cause the continental rift transformation within the oceanic rift matter. The plate-spearing process in mid-oceanic ridge zones is followed by the mechanism of creating a new oceanic crust happening under the influence of the magmatic basaltic melt. In the process of plate-spreading, every single extension impulse is backed up by the supply of an additional matter of mantle melt. If the issue is solidified, it can strengthen the plate edges that diverge from the mid-ocean ridges’ axis. The formation and creation of new oceanic crusts are taking place precisely.
In case an oceanic plate and continental one collide with each other, such a phenomenon as the subduction happens due to merging the heavier plate (oceanic) with the continental scale. In other words, when two oceanic plates come into collision, the older and cooler scale is immersed into the newer plate that is less dense. Wang Wenzhong et al. (2020) study suggests that “the presence of subducted oceanic crust could provide good explanations for some lower-mantle seismic heterogeneities with different length scales” (1). Subduction areas are adjusted to the axial zones of deep-sea currents that are correlated to island arcs.
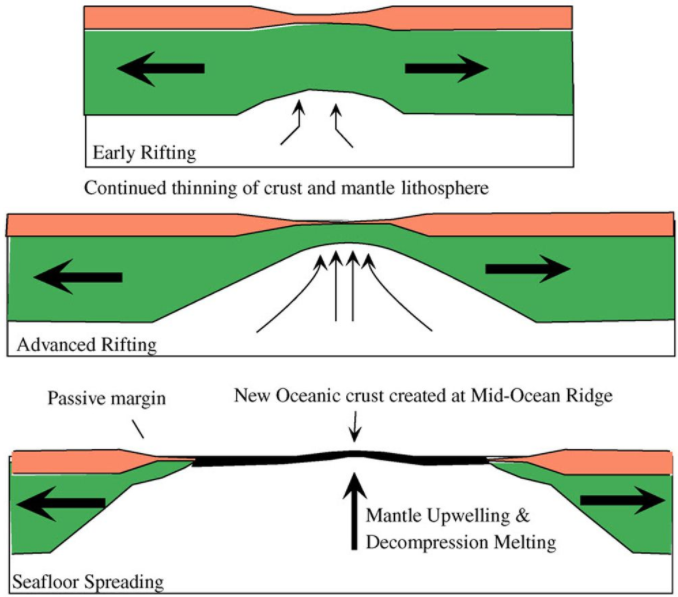
These specific zones are characterized by the particular structure, where significant components are back-arc basins, volcanic island arcs, and deep-sea trenches. As to an oceanic trench, it is formed in the curved bent in the subducted plate. The more it sinks further, the more water the subducted plate loses. This plate lacks water minerals and sediments, which causes the reduction of the melting indications in the core structure. Jones et al. (2020) state that “long-term oceanic crust formation and recycling leads naturally to the formation of relatively dense thermochemical piles in the lowermost mantle” (10). There might be some stretching in the back of the volcanic arc; the extension sets a back-arc basin formation.
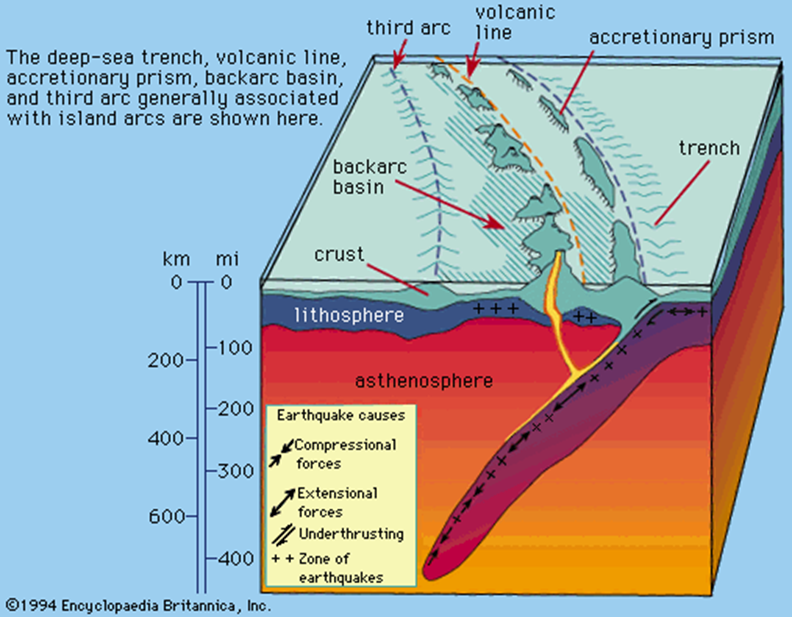
The largest number of volcanoes is located on the Pacific Ocean periphery, forming the so-called “Ring of Fire”, which is confined to the active continental margins. Island arcs and the North and South American Cordilleras lie on these margins – structures separated from the ocean by deep-sea trenches. There, inclined zones are traced from the trenches towards the continents, within which the foci of numerous earthquakes are located. Such zones are labeled as seismic focal zones; they interact with lithospheric plates causing the possibility of earthquakes and volcanic activity.
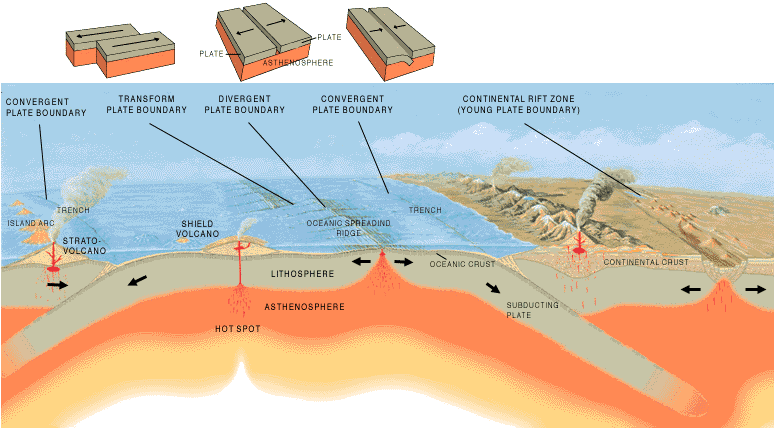
The subduction of oceanic plates under the continents, if it is not compensated by their expansion in the mid-ocean ridges, usually leads to a gradual closure of the ocean. This process is also accompanied by a collision of the continents that frame it, as well as the occurrence of a collision fold belt along the moved-under zone of the plate. A credible example of that is the Alpine-Himalayan mountain belt, which arose on the site of the ancient ocean Tethys. The process of plate thrusting continues to this day, which is evidenced by the increased seismicity of this region. Therefore, the Alpine-Himalayan mountain belt can also be considered a convergent or collisional border of the plates.
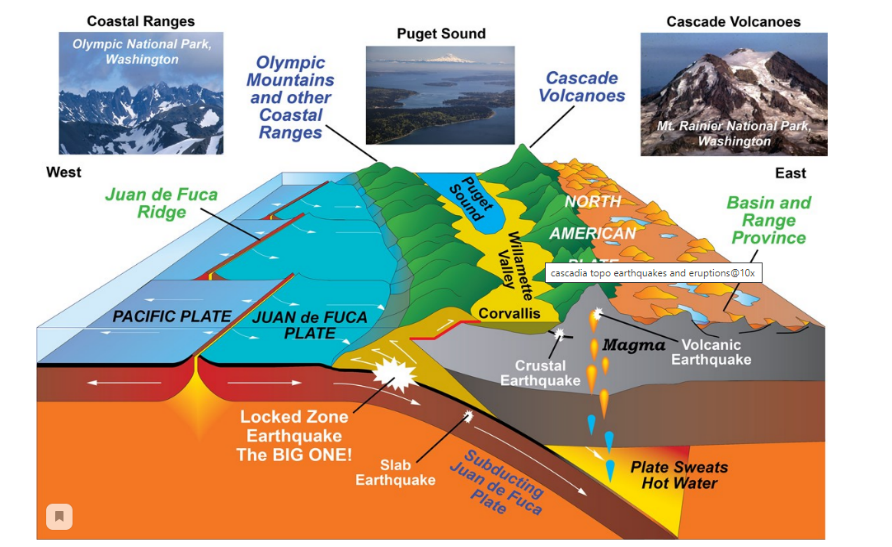
Dark Colored (Mafic/Basaltic) Igneous Rocks
Basalts formed in different geodynamic settings quite often bring to the surface deep mantle xenoliths. These formations contain information on the composition and conditions of existence of mantle rocks. Study of peridotite xenoliths found in the provinces of modern suprasubduction volcanism is extremely relevant for comprehending the overall structure of the lithospheric mantle and reconstruction of processes occurring over subduction zones.
Olivine – The Green Peridot
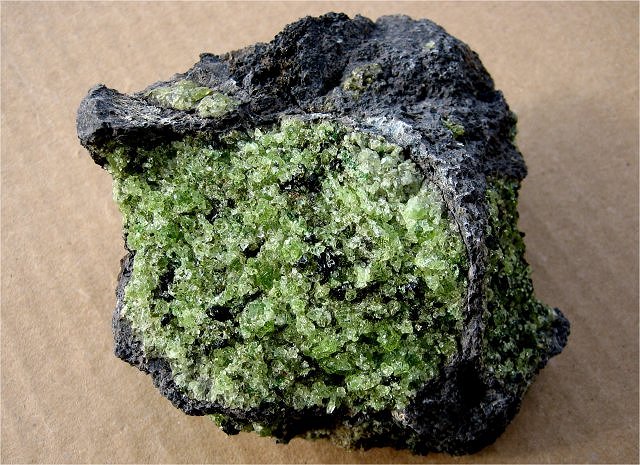
Olivine is a mineral, magnesium-ferruginous island silicate from the peridots group. At present, the name refers to the isomorphic series of forsterite-fayalite with the general formula (Mg, Fe) 2 [SiO4]. The content of Fe and Mg varies between two end members: forsterite with the formula Mg2 [SiO4], and fayalite – Fe2 [SiO4]. The color range of olivine varies from colorless to black, yellowish green, olive and bottle green. The mineral’s hardness is around 6.5-7, and the specific gravity is 3.27-3.37 g/cm3. There are continuous granular masses or crystals and grains usually included in the rock. In rocks, the mineral forms irregularly shaped grains with a strong glass luster, its inner structure is rhombic. The characteristic form of olivine separation is the hexagonal or diamond-shaped crystals.
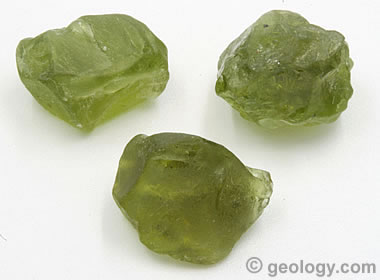
Olivine composes basic and ultrabasic effusive igneous rocks, and is very widespread in the mantle: the mineral is present in kimberlites (diamondiferous rocks), komatiites (ancient high-temperature lavas), and basalts. It is usually created due to the differentiation of magmas, and is one of the first to emerge, being one of the richest minerals on Earth. Olivine can be often found in contact marbles and magnesium skarns, as well as in regional metamorphisms and metasomatic formations associated with intrusions of ultrabasic and alkaline rocks. Under the action of hot aqueous solutions coming from magma chambers, olivine transforms into serpentine or talc. This process, known as serpentization, is very characteristic of olivine-bearing rocks.
Pyroxenes
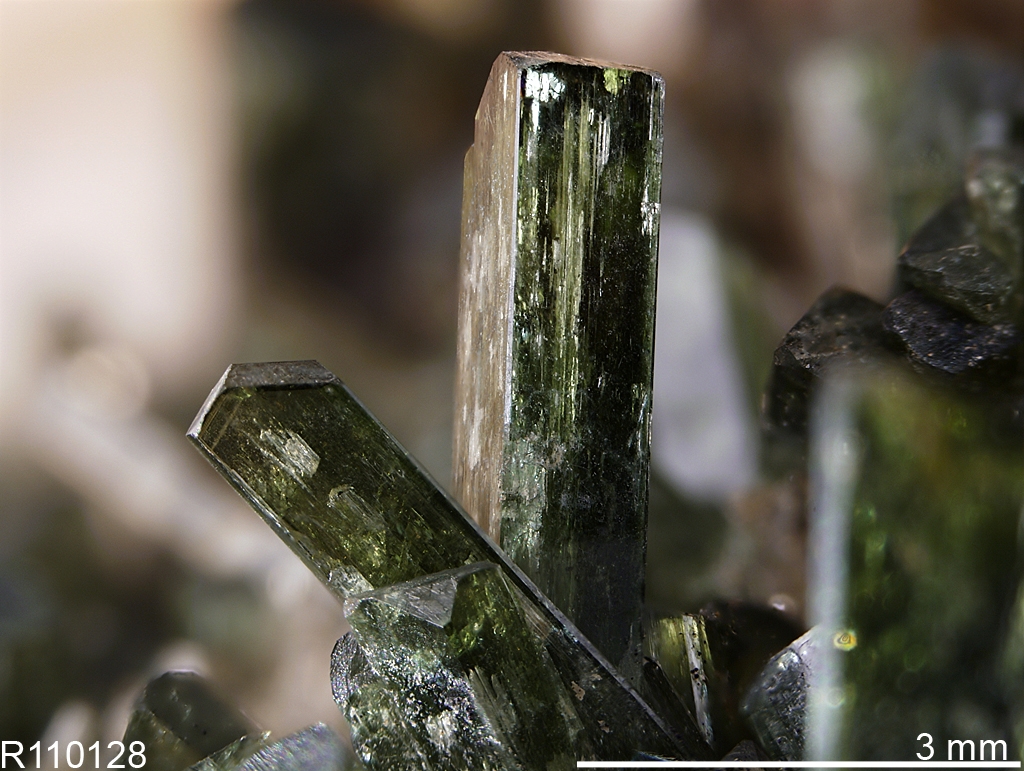
Pyroxenes is a group of rock-forming minerals of the subclass of chain silicates. They form prismatic crystals with an octagonal cross-section, columnar and radial-radiant aggregates, confluent fibrous and granular masses, or disseminated grains of white to brown and black. However, most pyroxenes are green in various shades. A perfect cleavage along the prism at an angle of 90° for orthopyroxenes, or about 87° for clinopyroxenes, is very characteristic. The mineral has the rate of hardness of 5-6, with density around 3100-3600 kg/m3. Magnesian orthopyroxenes are found in many ultramafic rocks (peridotites, picrites, some pyroxenites), and mafic rocks (norites), as well as various metamorphic rocks of the granulite facies. When it was established that igneous rocks of basic composition are widespread in the Earth’s crust, it turned out that pyroxenes are one of the main components of these rocks.
Thus, pyroxenes are high-temperature intrusive minerals that are part of igneous and deepest metamorphic rocks, as well as skarns. Spodumene is a characteristic mineral of granite pegmatites, while aegirine and aegirine-augite are alkaline rocks. Pyroxenes are typical chain silicates: their crystal lattice is built of simple single chains of silicon-oxygen tetrahedra, between which cations of metals are located. These crystals have a prismatic appearance – from short-prismatic in diopside to acicular in aegirine, but always with a clear elongation in one direction. This is an extremely characteristic feature of pyroxenes, distinguishing them from similar and equally widespread amphiboles. In cross-section, pyroxene crystals have a rectangular, almost square, or octagonal shape, which also distinguishes them from amphiboles.
The color of pyroxenes is determined mainly by the amount of iron in them. Usually it is dark green to black, but sometimes it lightens up to white or almost colorless such as in diopside or spodumene. Some varieties of spodumene are even colored pink, lilac or green due to impurities of manganese or chrome. The luster of pyroxenes is usually glassy, sometimes pearlescent – in spodumene, for example. During hydrothermal alteration, they are replaced by serpentine, uralite, or an aggregate of epidote and chlorite. When weathered, they decompose into iron hydroxides, opal, and clay minerals.
Vesicular Basalt
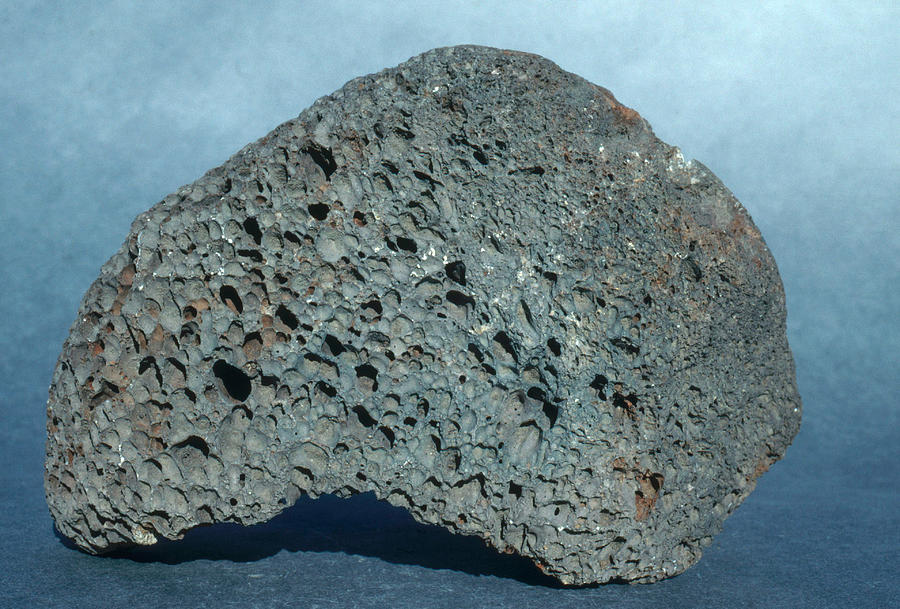
Basalt is a kind of volcanic rock formed under the influence of basaltic lavas. According to the chemical mineralogy assessment, vesicular basaltic rock is supposed to be a natural and effusive stone typical of gabbro. This rock is effusive, the main minerals contained in this rock are clinopyroxene and phenocrysts. Some swellings formed in the upper part of the basaltic lavas were created under the influence of the evaporations of gas elements and water. These swellings are a place where some minerals, such as native copper and calcite, might be gathering. These formations of minerals entail the process of amygdaloidal basalt creation. Particular components of vesicular basalt rocks are so tiny that they can be observed with the help of a microscope. Vesicular basalts possess a porphyry structure that enables an observer to view all crystal-line components in them. Visible crystal components embrace porphyry phenocrysts formed from augite or plagioclase.
Very often, vesicular basalt consists of the same minerals, which are glassy magnetite, having a crystocrystallinized porphyry or aphyric structure. Referring to the porphyry variations, small phenocrysts of isometric crystals that are greenish-yellow or olivine are visible due to black pyroxene prisms. The phenocryst size is about several centimeters in length, and it is 20-25% of the rock mass. The vesicular basalt texture and structure might be massive, dense, porous, and amygdaloid. The vesicular basalt currents are detected by the columnar jointing transpiring because of the process of rock cooling. When it comes to marine basalts, they are formed due to the rapid cooling of the lava flow surface merging with water.
Vesicular basalt is the most common effusive rocking matter in the Universe. The basalt bulk is created in mid-ocean ridges and the process of oceanic crust formation. Additionally, vesicular basalts are typical environments full of rifting, active continental margins, and magmatism. During the formation process of crystallized basaltic magma, layered instructions such as the Bushveld and Norilsk are formed. The color scheme of this effusive rock ranges from a dark gray to a greenish-black tone with a glassy structure. As a rule, the color range of basalt is narrow; it is rich in a distinctive black shade. The design of vesicular basalt is considered to be glassy and well-grained.
Scoria
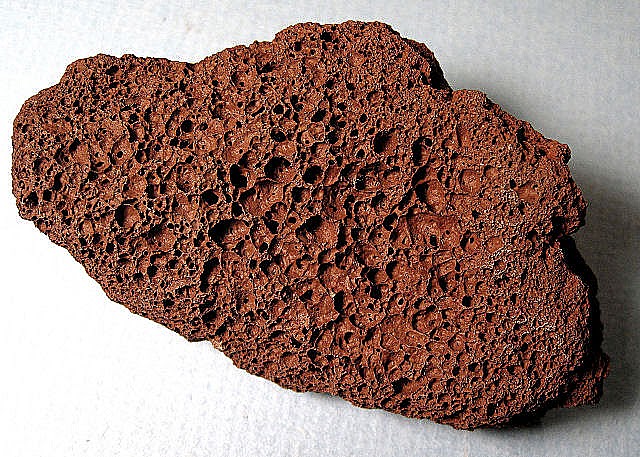
Scoria is an effusive volcanic large-porous loose rock with a spongy structure and dark color, consisting of volcanic slags. Unlike pumice, scoria is formed from more fusible magma, which had a more liquid consistency during the eruption. Scoria cones on the sites of volcanic eruptions can reach 1000m in base diameter, and height up to 260m, with sediment volume up to several million cubic meters. Thus, scoria deposits sometimes occupy tens of square kilometers with a capacity of up to several tens of meters.
In regards to basic structure, scoria is a loose pyroclastic mixture of sand and gravel. Its color varies widely: from purple, red, and orange to yellow, pink, brown, sometimes gray, and black. Scoria’s fragmented porous structure can reach up to 40% of vesicles rate, due to which it has a low thermal conductivity. Moreover, scoria is largely characterized by the fact that, against the background of a mass with a porous structure, fragments of various sizes, different shapes and different colors are scattered. Thus, it is concluded that the rock is heterogeneous. The specific gravity of scoria reaches 1.4-2.5 g/cm3, with compressive strength up to 60 MPa, and Mohs hardness rate of 3.
More than half of the chemical composition of this rock is formed by silicon oxide and aluminum oxide. In addition, the composition of the scoria includes oxides of iron, magnesium, potassium, sodium, and other chemical elements in minor amounts. It happens because of fact that scoria is created by mixing liquid lava with sand particles and volcanic ash. Physical and mechanical characteristics might fluctuate in relevance with the type of stone. For example, the degree of sintering, depending on which caked and cemented varieties are distinguished, determines the strength parameter.
Light Colored (Felsic/Granitic) Igneous Rocks
Granite
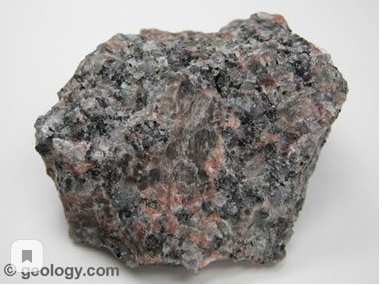
Short Description: coarse-grained (phaneritic), intrusive, light-colored/felsic crystalline igneous rock.
Granite is considered to be an equigranularity and felsic rock that has an intrusive and light-colored structure. Generally speaking, this rock is one of the oldest stone matters on Earth. Granite is famous for its abundant makeup full of sedimentary components covering most of the continents. This rock is formed in primary volcanic arcs, and the production of this rock is the result of the collision and merging of two continental substances or masses. The first-ever continental substances or masses were the outputs of the cumulation of the volcanic arc matters. It predisposes the location of granite in the cores of all-natural grounds. Granite is supposed to be the plutonic substitute for rhyolite.
- Color– generally light-colored;
- Texture– hard-grained (phaneritic);
- Mineral make-up– plagioclase, orthoclase, quartz, small quantities of biotite;
- SiO 2(Silica Content)- approximately 70%.
Obsidian
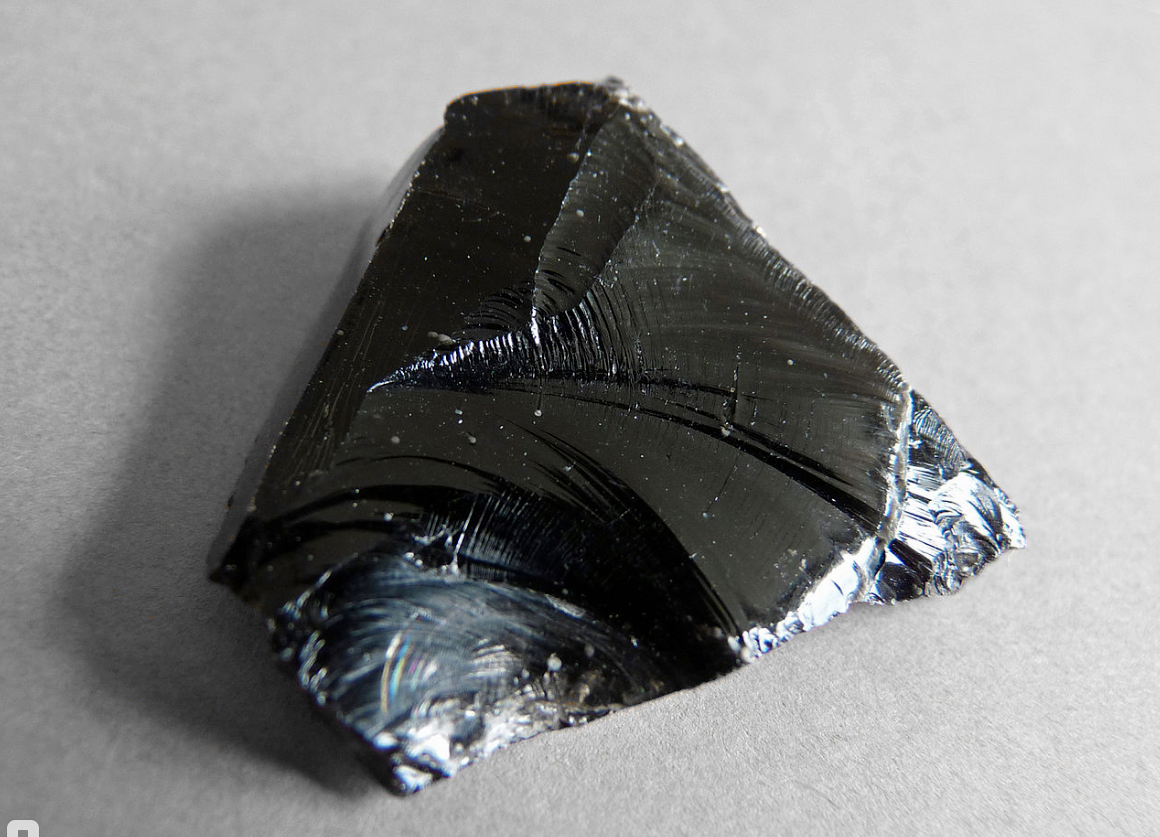
Short Description: felsic volcanic glass
Obsidian is an igneous rock existing as a natural glass formed during the rapid process of cooling of viscous lava occurring from volcanoes. This rock is abundant with silica (SiO 2), and silica content equals 65 to 80 %. Obsidian chemical make-up and composition can be compared to rhyolite, as they have similar components in their make-ups. Obsidian lacks water in its chemical composition, but it possesses a glassy shining. However, the obsidian color tone is characteristically jet-black. Iron oxide (hematite) is the primary component of the obsidian component, and it creates brown and red color variations. Small gas bulbs that are included in the obsidian contest might produce a golden luster. As to its water inclusion, it consists of only 1 % of water. The surface eruption, where pressure is not so high, prohibits the volatile water from fading away, and this process stimulates the increase of the melt viscosity. The increased level of density hinders the process of crystallization, as the lava hardens as a glass.
Pumice
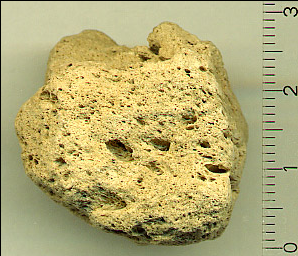
Short Description: glassy, felsic, igneous rock – frothy – 75-90% vesicles/ frozen bubbles
Pumice is a kind of volcanic rock primarily consisting of vesicular glass with a rough texture. This texture might be comprised of crystals, but also it might lack crystals. Typically, it is light-colored and has thin vesicle walls. Pumice is formed under the influence of the ejection of highly pressurized and superheated rocks from volcanoes. The foamy pumice format transpires due to rapid depressurization and cooling at the same time. The depressurization forms bulbs via declining gas solubility (embracing CO2 and water). These gases are disintegrated in the heat temperatures of lava, which makes these gases bar the regulating of gases. This process can be compared to the appearance of CO2 bubbles predisposed by an available carbonated drink. In the matrix, the bubbles are frozen through simultaneous depressurization and cooling. Tiny crystals of several minor minerals might be observed in most pumices; the most major are augite, zircon, feldspar, hornblende.
Silicate Minerals
Labradorite
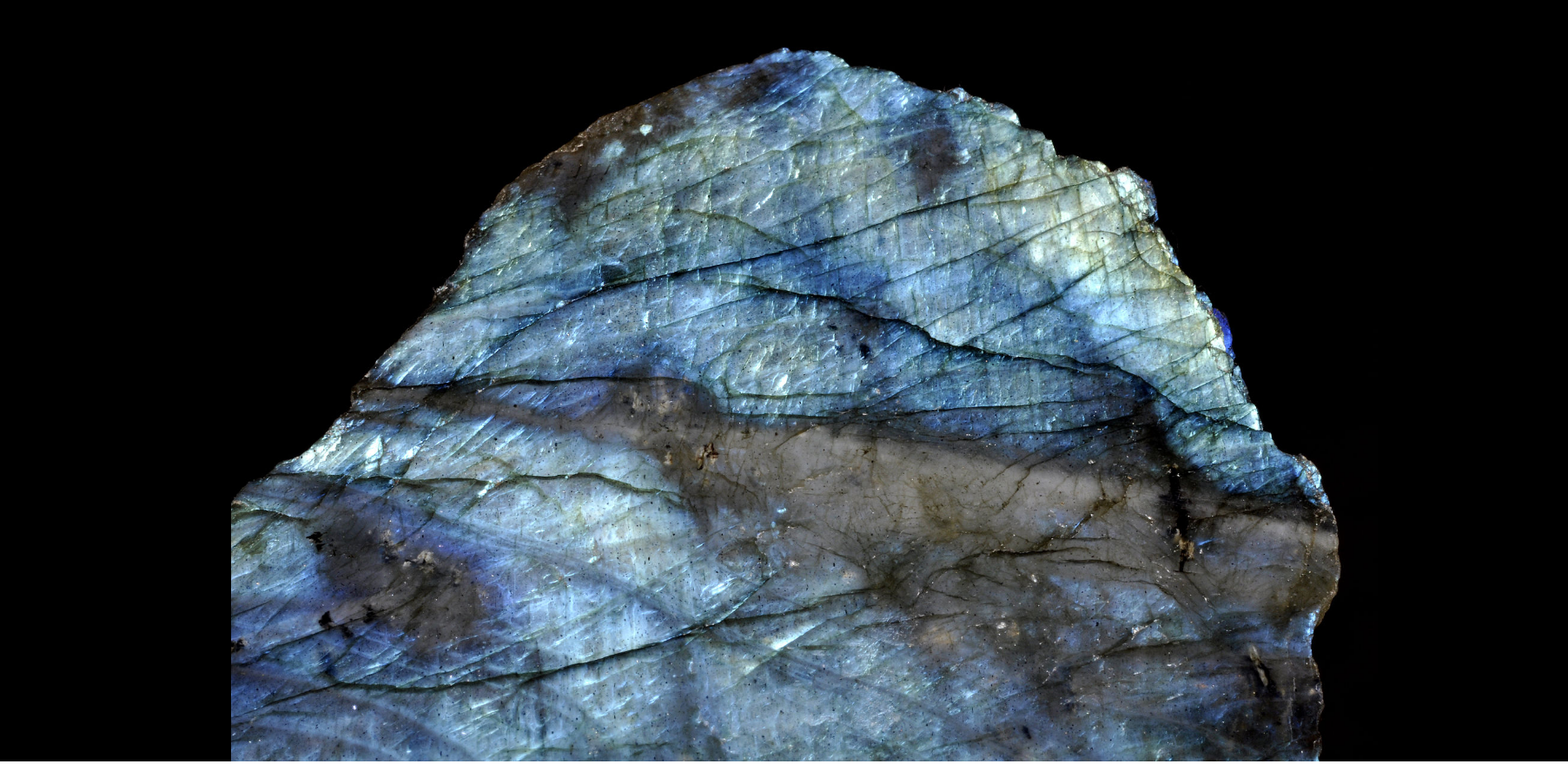
Short description: (CaNa)Al1-2Si3-2O8, with Na 30%-50%, Ca 70%-50%} – a sodium-rich plagioclase feldspar series – coarse-grained (phaneritic), intrusive, igneous rock; famous for the “labradorescence” (prismatic sheen) on the polished surfaces.
Labradorite is considered to be a significant mineral of feldspar matter. Feldspar rocks consist of about 40% of the mainland crust of the Earth in terms of evaluating the weight of feldspar rocks. The labradorescence issue in their make-ups can characterize labradorite rocks. It stimulates the creations of these stone shapes: tubular and thin. As to the color range, these stones have several tones, such as blue, green, grey, yellow, and sometimes brown. The crystals of these rocks have a serene pearly luster that creates the schiller effect. The gravity of this stone equals approximately 3%; it is a hard stone. In accordance with the Mohs of hardness, its hardness rates 8.
Mica
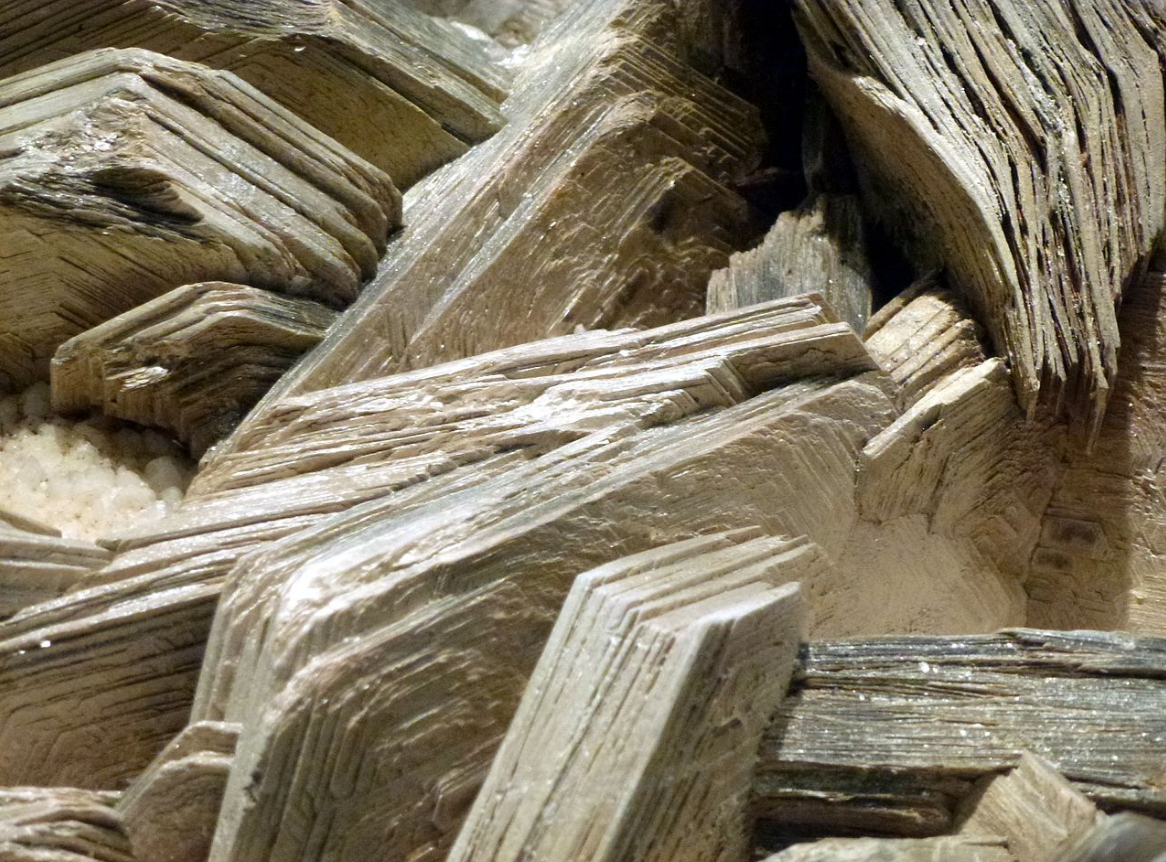
Short Description: coarse grained igneous or metamorphic rocks (AB2-3(X, Si)4 O10(O, F, OH)2) (mica series includes biotite (gold-brown), muscovite (white), lepidolite (purple), phlogopite (green).
Biotite, muscovite is commonly black and abundant with phlogopite (black-colored) and paragonite. Paragonite is an element that can hardly be observed macroscopically, as it is invisible. The most significant characteristic of mica is that its crystal structure can be easily split into elastic slides(plates). This rock is characterized by perfect basal cleavage. The rock solidity is the medium level as it can be easily disintegrated. In accordance with the Mohs of hardness, its hardness rates 6.
Milky Quartz
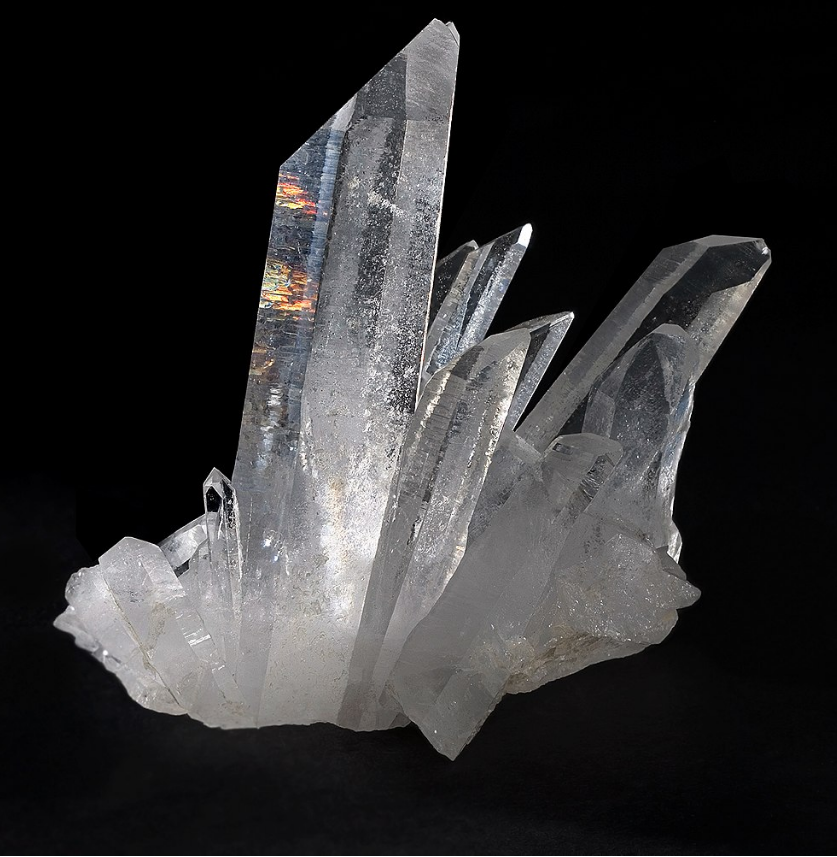
Short descriptions: SiO2, milky quartz (white, translucent to opaque).
Quartz is a hard and crystallized mineral consisting of silicon dioxide (silica). Behind feldspar, milky quartz is the second abundant mineral in the mainland crust on Earth. Milky quartz is chiral structured and exists in two variations: the high-temperatures and the regular quartz. Milky quartz is a semi-precious gemstone. In accordance with the Mohs of hardness, its hardness rates 7.
Mitosis and Meiosis
Mitosis
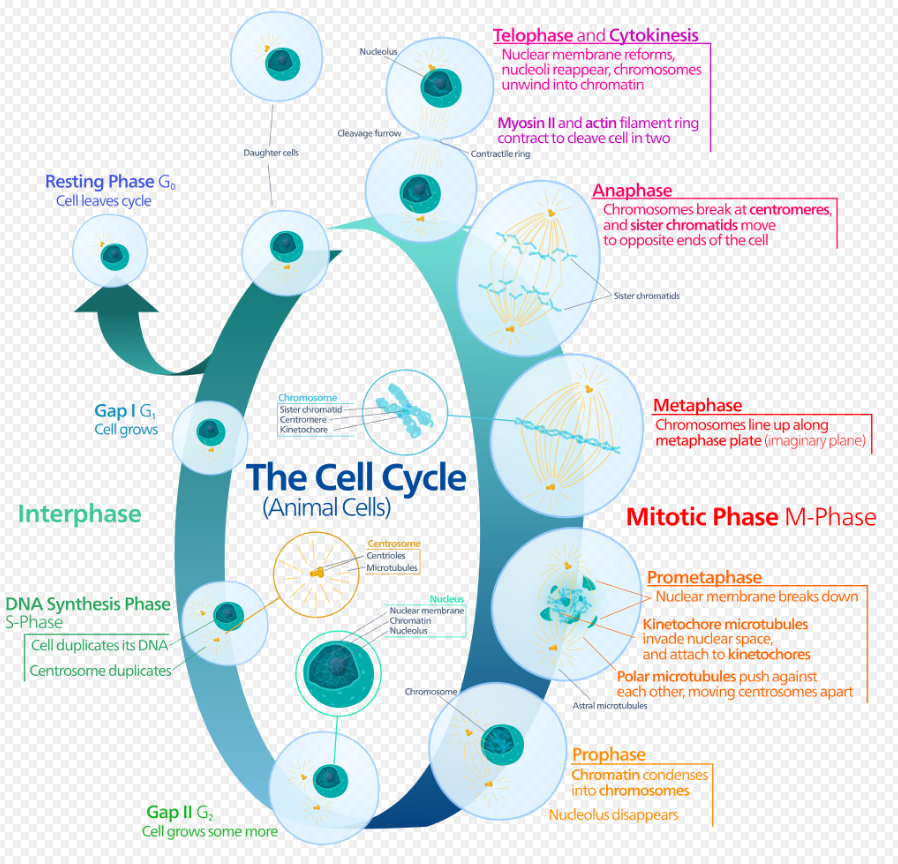
Mitosis is the process of cell reproduction or duplication while one cell stimulates the rise and development of two identical daughter cells. In this process, diploid somatic cells are produced as the result of replication of nuDNA and chromosome distribution; these structures have the genetic information of a cell. The divided cells that carry the data are the result of the merging of centromere and chromatids. As a rule, mitosis is characterized by the interphase of the S stage that is preceded by cytokinesis and prophase, metaphase, anaphase, and telophase.
Meiosis
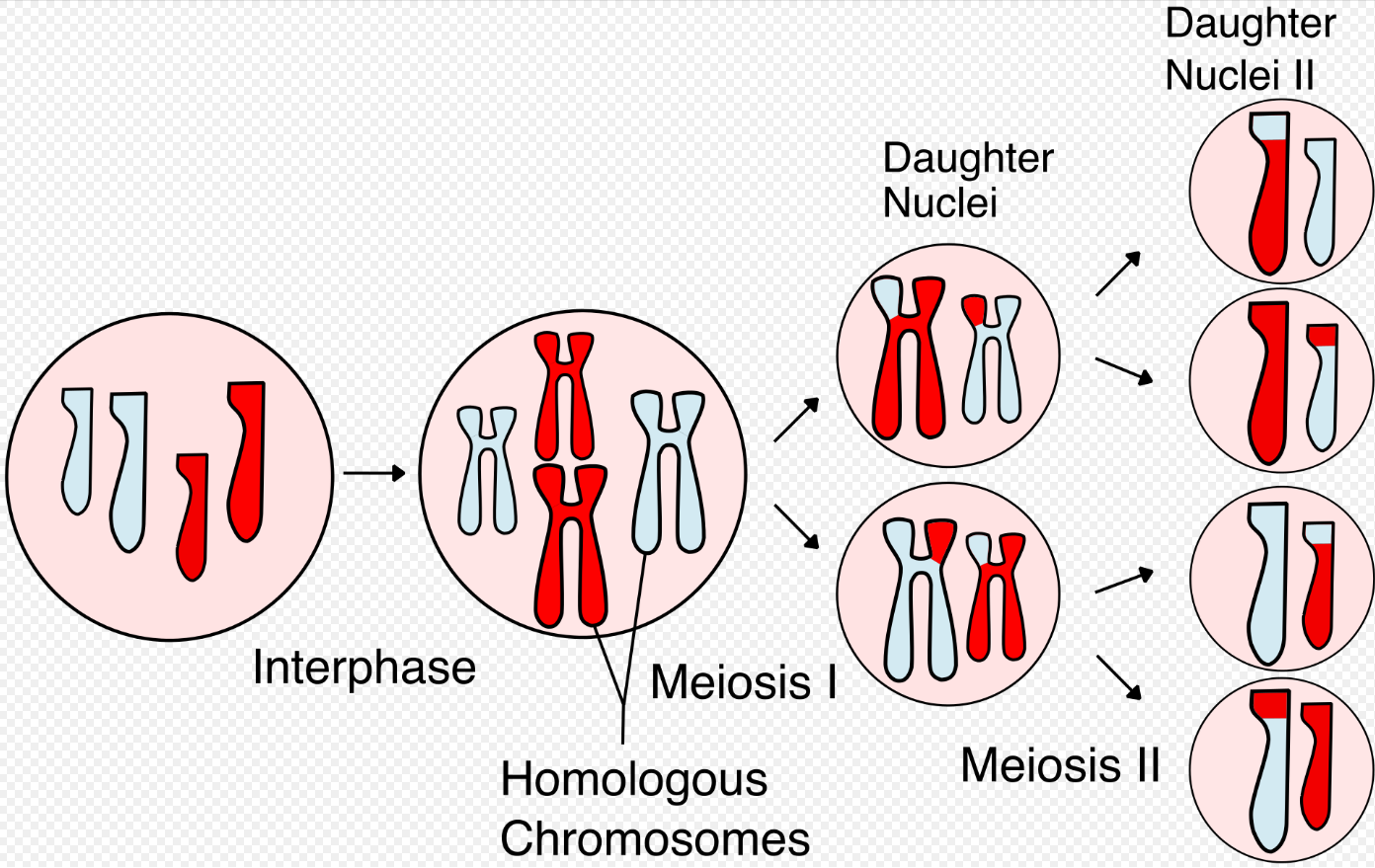
Meiosis is a kind of cell division of sexually reproducing microorganisms liable for haploid gametes production (egg cells and sperm). It is a case of two circles of cell division of parental cell copies that have homologous chromosomes in their make-ups (the process called synapsis). To form a new code, combinations of maternal and paternal copies of chromosomes cross over with each other. While fertilization, the haploid cells start to create diploid zygotes. In this kind of cell division, nuDNA replication is followed by two circles of cell division. Meiosis can have two subtypes, Meiosis 1 and Meiosis 2, that can be divided into Prophase 1, Metaphase 1, Anaphase 1, Telophase 1, and cytokinesis for Meiosis 1. The same components (Prophase 2, Metaphase 2, Anaphase 2, and Telophase 2 and cytokinesis) are typical of Meiosis 2.
DNA Replication
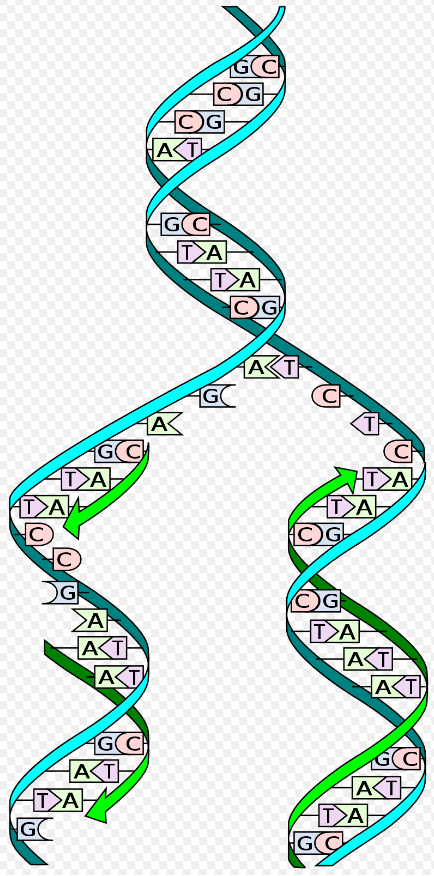
DNA replication is the process when DNA molecules(double-stranded) are divided to create or produce two similar DNA molecules. DNA replication is supposed to be the most typical basis transpiring within cells. Every time a cell is split or divided; two daughter cells produced via its division possess DNA (the genetic information). The genetic information daughter cells carry is the same as parental cells have. DNA replication transpires in three significant steps. At the beginning of cell division, the DNA separation and the double helix strand are produced. After it, the separation starts, and DNA polymerase (enzyme) stimulates the production of the new DNA strands. Two steps characterize the beginning of DNA replication. The first step occurs when protein dismantles a short DNA double helix stretch (short sequences of DNA nucleotides are called Okazaki fragments). Then this protein (helicase) starts to affix to and disintegrates to create RNA primers. While the helicase moves toward the DNA molecule, it starts to split the two nucleotide chains. The nucleotides forming new strands are attached to partner nucleotides in the sample strand.
This biological phenomenon is called complementary base pairing, entailing the formation of the DNA complementary strands. Pairing structure is the basis of a nucleotide sequence located in the current sample strand attached to a new sequence called an anti-sequence of the recent sample strand. Further, after the new strand is replicated itself, its new strand will embrace the typical sequence of the current sample strand (LCR- ligase chain reaction). In this case, the DNA replication occurs in several sequence series and anti-sequence series that copy and duplicate the original DNA codes. Polymerases of DNA duplication lead DNA to the 5’ and 3’ direction, where the DNA helix is antiparallel.
DNA and Protein Synthesis
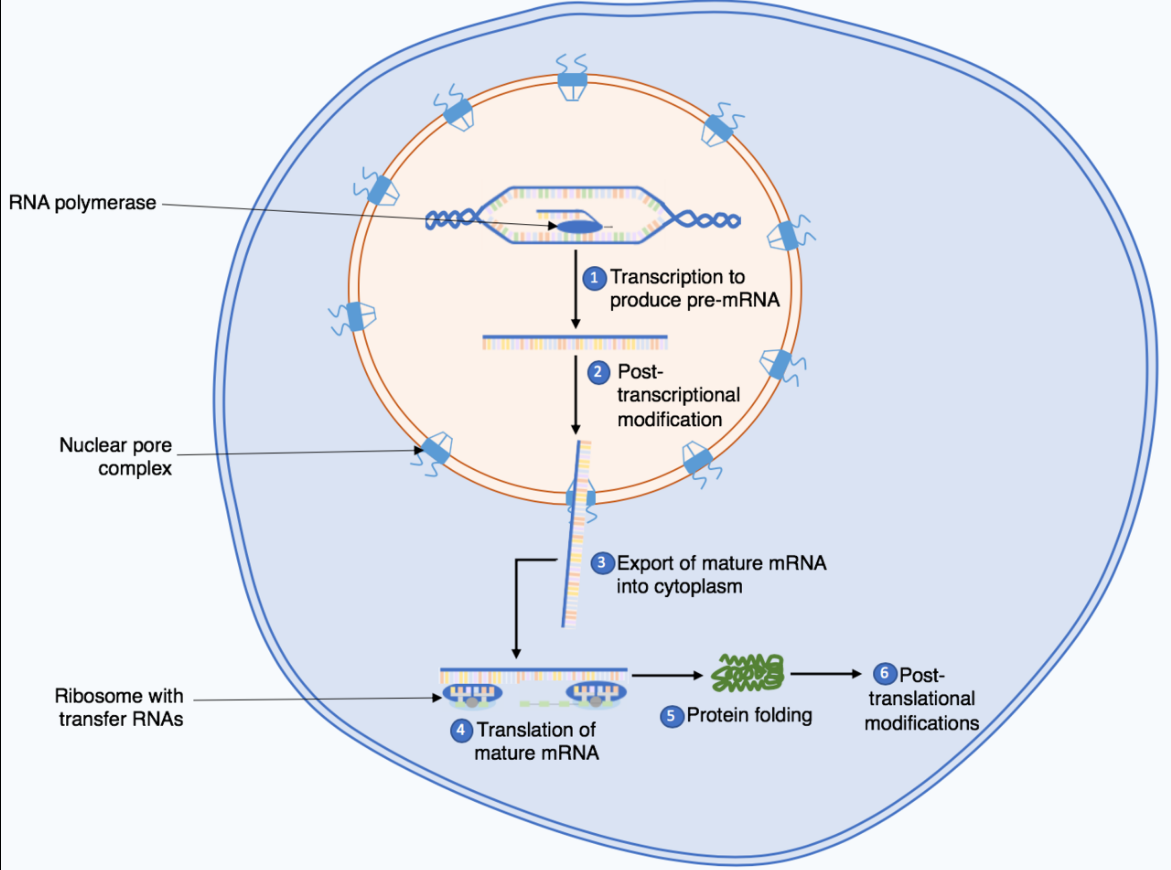
Protein synthesis occurs in cells of every living being; this process embraces two significant functions, such as translation and transcription. Transcription occurs in the nucleus of eukaryotic cells. During this transcription, DNA is some sample or template to modify a molecule as a messenger RNA and nuDNA (mRNA). The mRNA molecule stimulates the translation process when it moves to a ribosome. The biological scheme of protein production and its code is DNA → RNA → Protein.
The translation is the other significant part of the protein production, as this process is liable for mRNA output responsible for producing protein. When mRNA comes from the nucleus position, it goes towards a ribosome. The ribosome repeats the pattern of mRNA codons and carries amino acids in the correct ribosome sequence. The structure of tRNA molecules possesses an anticodon that has amino acids with its construction. An anticodon is considered to be the codon basis for amino acids. Further, lysine is carried and transported by tRNA molecules. tRNA combined with the amino acids creates a protein or polypeptide chain. In protein synthesis, the leading role goes to the process of ER creation (endoplasmic reticulum), which is liable for eukaryotic cell transportation.
Fossil Stromatolites, Charnia
Fossil Stromatolites
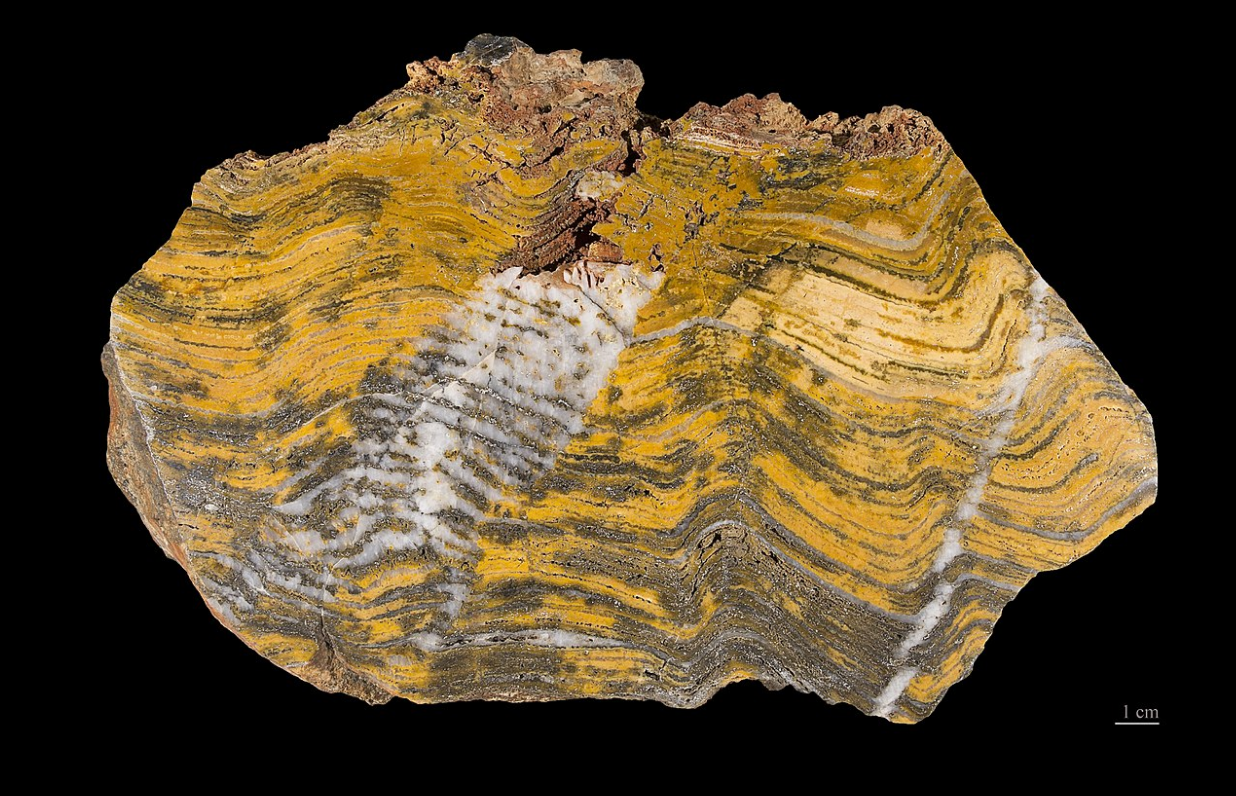
Stromatolites are supposed to be the oldest living fossils existing on the Earth. Its original identity derives from the Greek “stroma,” implying lithos or mattress. Stromatolite means rock, referring to the Solar System origins. According to scientists’ observations, stromatolites are characterized as the stony or rocky matter constructed with the aid of using sets of photosynthesizing micro-organisms known as cyanobacteria. In contrast, hard sediments go deeper in layers of shallow water. Micro-elements start to emerge above it, mixing the constructing layer and debris that finally become barrows. Residing stromatolites are decided in solely salty lagoons or bays on the planet. Western Australia is a common place for its range of stromatolite sites; each of them is residing and fossilized. Fossils of the earliest stated stromatolites are approximately three-layered. Five billion-year-old antiques are placed about 1,000km north, close to a marble bar within the Pilbara area.
Charnia
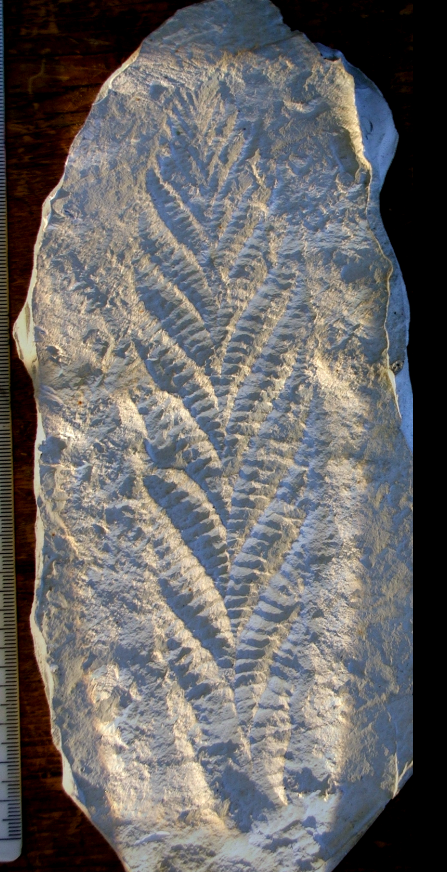
Charnia is a genus Ediacaran lifeform with a zig-zag structure branching in the leaf-like ridges. Charnia is absolutely significant due to its first Precambrian fossil to be discovered. Charnia is supposed to be the oldest life form ever existing on the Earth. While resembling the fern that was obtained from an alga and sea pens, Charnia was a different and not typical form of life back then. The Charnia discovery marked for the first time when diverse living organisms were found in the rock matters. Those discoveries were aligned with finding fossils in all materials. While the discovery of deep-sea bottoms, Charnia were found, and it presented fossil beds. Fossil bed evaluations where Charnia has been discovered made scientists conclude that Charnia inhabited deep waters that sunlight was unable to reach that set point. Under the elaborated assessments, there has been a conclusion that Charnia consisted of latticework tissues.
The Burgess Shale Fossil Fauna
Hallucigenia
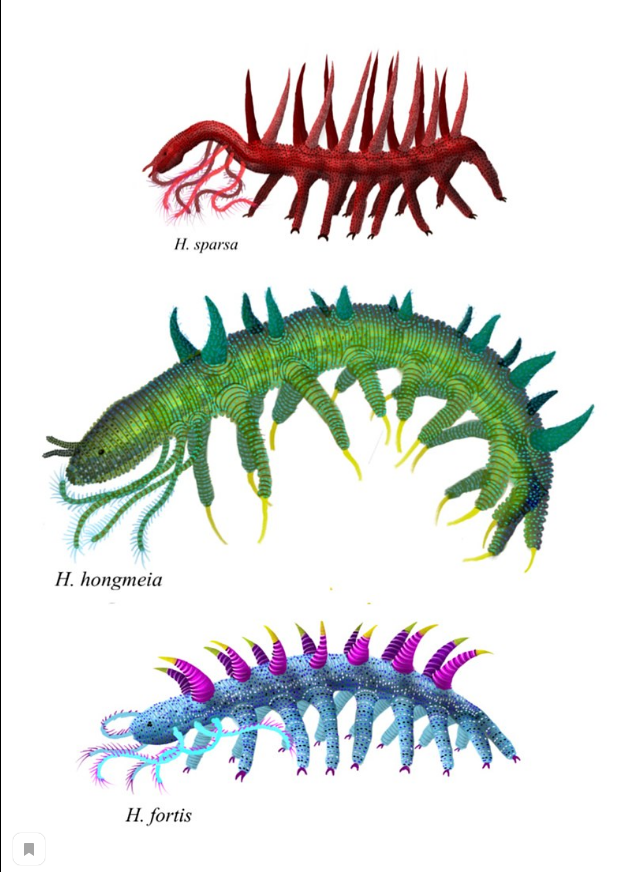
In the 1970s, the freakish Hallucigenia was first discovered, and paleontologists were unable to find and distinguish its tail from the head. The weird creature is the ancestor of living molding creatures such as worms, crabs, and krill. While assessing that creature, enormous spikes on its back were discovered. This animal lived 500 million years ago in the period of the Cambrian explosion (the time of the excessive boom considering the variations of evolution and life). This creature was primitive and advanced in its age. The fossil sediments of this creature were first found in Canada, and it looked like a worm with spikes and legs. Hallucigenia is the representative of a group known as ecdysozoans embracing onychophorans (velvet worms) and tardigrades (water bears).
Wiwaxia
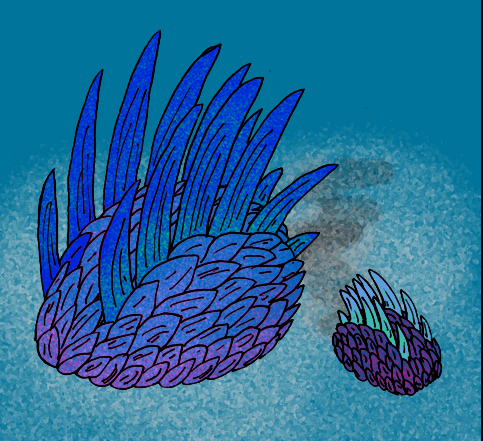
Wiwaxia was found in 1899 and described as a creature having dorsal spines. Wiwaxia was the representative of a polychaete worm having a squat body in the elliptical form. It was reminiscent of a small squashed circle. There were tiny protuberances around its body that resulted in the forming of its sides possessing upper lateral portions. There was no distinguished growth that facilitated the creation of a head. The sclerites covering the Wiwaxia body formed typic armor with ridged and sharp scales. The sclerite root was approximately 40% of the anchored tissue into a pocket of skin. The sclerites acted out as defense and reliable protection from the suction kinds of teeth other animals got.
Opabinia
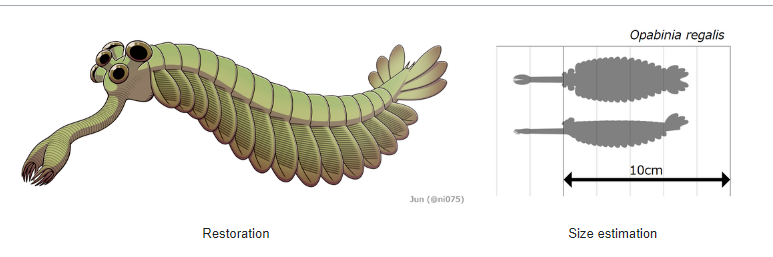
Opabinia is characterized as a loop with an arranged in segments body; these segments are labeled as lobes. Opabinia has a soft body with no exoskeleton (arthropods later formed in this exoskeleton). As a rule, the smooth body is destroyed during the process of preservation, entailing less significant differences in reconstructions. Due to the softness of the body, Opabinia’s internal organs are uncovered in slight impressions. It means that only an experienced and proficient eye is unable to identify. And detect these micro organs. The lobes of the main body are flat and situated in the projection of the body downwards curves and angles. As a rule, these lobes are attached to each other and rest over the lobe’s front edge. In case the gills are situated beneath the lower side of the lobes, they can be protected. The Opabinia’s lobes create the form of “V,” and they stick to the tail; these peculiar features make Opabinia closely reminiscent of a jet.
Marine Arthropods: Paleozoic Trilobites, Extant Horseshoe Crabs
Paleozoic Trilobites
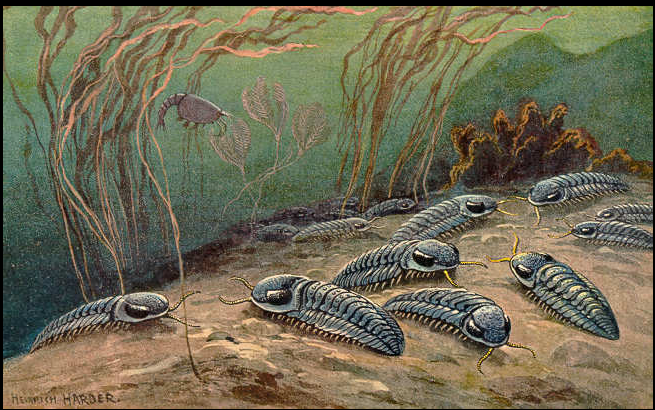
Trilobites are considered to be the earliest animals on the Earth; it is the form of fossil existing on the Earth. They appeared in the Cambrian Period and primarily inhabited the Ordovician and Cambrian seas. It is a kind of invertebrate living creature embracing insects, spiders, and crustaceans. Trilobites are representatives of arthropods presenting the phylum Arthropoda class. Scientists supposed these creatures to live in deep marine waters on the rocks and reefs. While examining trilobites, scientists proved the fact that they presented fossils. For protection and support, exoskeletons cover the soft parts of a body. As a rule, the dorsal part that is located on the back was full of fossils. The underside (ventral) part of a body was covered by minerals of a thin membrane that were not fossilized. The exoskeleton was divided into a central axis where two side lobes were located (these lobes were called pleurae). The pygidium, thorax, and cephalon are split into particular segments. The central part of the cephalon is called the glabella. The glabella cheeks are transferred utilizing a facial texture separating cheeks for the cephalon (on the outer edge).
Extant Horseshoe Crab
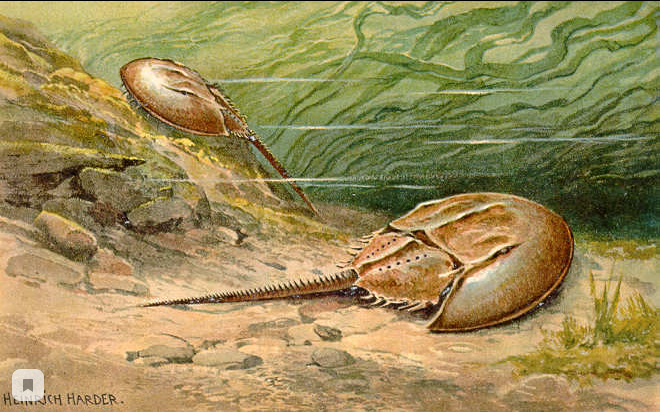
Extant horseshoe crabs are the representatives of diverse chelicerate arthropods that are included in the Limulidae family. Horseshoe crabs are Limulus species of the Atlantic waters. Horseshoe crabs typically have a domed, horseshoe-shaped, and heavy carapace; a spiky and pointed tail (telson), compound eyes, and a mouth with no jaws. Despite the given name, these crabs are related to scorpions, spiders. Under their carapace, they are reminiscent of spiders. Large fossil amounts characterize these creatures, and these long-existing living animals have not changed their forms and shapes for 445 million years. In terms of ecological facilities, crabs are vital for marine and water food chains. They eat invertebrates, such as worms, crustaceans, and mollusks. Approximately 20 species of seahorse birds are in close connection with the eggs of horseshoe crabs. In medicine and research, horseshoe crabs are essential, as their micro components are used for improvement and healing people’s immune system issues. Extant horseshoe crabs have Limulus in their make-ups; Limulus helps investigate human beings’ vision issues. Nowadays, there is a decreased number of alive horseshoe crabs; the lack of these crabs might negatively impact the environment.
Works Cited
Albury, Donald et al. “The Specimen is a Cast of the Holotype of Charnia Masoni.” Wikipedia, 2021.
Appleyard, Anthony,“Evolution of Hallucigenia.” Wikipedia, 2021.
Avery, William et al. “Mitosis in an Animal Cell.” Wikipedia, 2021.
Bell, Trevor, and David Liverman. “Earth’s Mantle in Motion.” Heritage: Newfoundland & Labrador, Heritage: Newfoundland & Labrador, 2021.
Budge, Kent, et al. “Fossilized stromatolite.” Wikipedia, 2021.
Budge, Kent, et al. “Milky Quartz.” Wikipedia, 2021.
Budge, K., et al. “Paleozoic Trilobites.” Wikipedia, 2021.
Budge, Kent, et al. “Obsidian.” Wikipedia, 2021
Budge, Kent, et al. “Pumice.” Wikipedia. 2021.
Cross, Philip, et al. “Mica.” Wikipedia, 2021.
“Divergent Plate Boundaries.” Pixelrz, 2021.
Doubek, Joshua., Wikimedia Courtesy. “Seafloor Spreading”. National Geographic, 2021.
“Features of a Typical Island Arc.” Encyclopædia Britannica, 2021.
Geology. “Granite”. Geology.com, 2018.
Harder, Heinrich. “Extant Horseshoe Crab.” Wikipedia, 1916.
Jones, Timothy D., et al. “Subducted Oceanic Crust as the Origin of Seismically Slow Lower-Mantle Structures.” Progress in Earth and Planetary Science, vol. 7, no. 1, 2020, doi:10.1186/s40645-020-00327-1.
Joyce, A. B. “Vesicular Basalt.” Fine Art America, 2018.
King, Hobart M. “Green Peridot (Olivine).” Geology.Com, 2009.
Learning Geology. “Scoria.” Learning Geology, 2015.
Lillie, Robertson. “The Formation of a Coastal Mountain Range.” Oregon’s Island in the Sky: Geology Road Guide to Marys Peak. 2017
Lowell, Jack. “Diopside R110128.” RRUFF, 2021.
Nash, Scott. “Simplified Map of Earth’s Principal Tectonic Plates, Which Were Mapped in the Second Half of the 20th Century (Red Arrows Indicate Direction of Movement at Plate Boundaries).” Wikipedia, Wikipedia, 2006.
Reding, Tom. et al. “Labradorite.” Wikipedia, 2021.
Reding, Tom et al. “Mitosis in an Animal Cell.” Wikipedia, 2021.
Reding, Tom et al. “Protein Synthesis.” Wikipedia, 2021.
Sabo, Michael Joseph. “San Carlos Olivine & Peridot Minerals.” Geo Forward,2021.
Smith. “Plate Tectonics Matching Pictures.” Quia, 2020.
Vigil, Jose F. “A Cross Section Illustrating the Main Types of Plate Boundaries.” USGS, U.S. Geological Survey, 2016.
Wang, Wenzhong, et al. “Velocity and Density Characteristics of Subducted Oceanic Crust and the Origin of Lower-Mantle Heterogeneities.” Nature Communications, vol. 11, no. 1, 2020, doi:10.1038/s41467-019-13720-2.
Wikipedia. “DNA Replication.” Wikipedia, 2021.
Wikipedia. “Reconstruction of the Appearance of Opabinia.” Wikipedia, 2021.
Wikipedia. “Reconstruction of the Appearance of Wiwaxia Corrugata.” Wikipedia, 2021.