The selected asset in the environmental industry is the Photovoltaic Module (PV). It can be categorized as belonging to the Clean Energy Sources and Power segment of the industry. The PV Module is an asset of great significance, as it provides a possibility to convert the solar power into electricity, thereby contributing to the preservation of the environment. The report presented below outlines the management activities associated with the selected asset, identifies an approach to life-cycle cost analysis, as well as specifies the benefits of conducting life-cycle cost evaluation within the life-cycle management of the selected asset.
Asset Engineering Management Activities and Decisions
The PV Module is an essential piece of equipment in the innovative systems of energy production from direct solar radiation. In order to decide on the most suitable engineering asset management (EAM) decision, it is important to consider certain aspects of the EAM. Engineering asset management is a multifaceted process that requires analyzing several essential aspects, such as spatial generality, time generality, measurement generality, statistical generality, and organizational generality (“What is engineering asset management?” 2010).
Spatial generality refers to the human resources engaged in asset management, while the aspect of time generality distinguishes between long-term (lifecycle) and short-term (usage) dimensions of the asset. Measurement generality pertains to the value estimates, statistical generality provides an overview of potential risks, and organizational generality refers to the multi-level character of the EAM practice. Overall, it should be noted that engineering asset management is of a complex character.
All the mentioned aspects entail a joint input of professionals from various disciplines, such as engineering, economics, IT, and management (“What is engineering asset management?” 2010). Moreover, due to the character of EAM, the decisions within its framework vary from operational decisions in asset management to strategic decisions, pertaining, for instance, to a life-cycle design. The significance of qualitative data regarding human resources, despite the quantitative approach being the principal method in engineering asset management, is also emphasized (“What is engineering asset management?” 2010).
In order to identify a key engineering asset management decision, it was necessary to analyze the aspects of engineering asset management. Moreover, it is crucial to describe the basic engineering management activities associated with the management of the PV Module in the environmental industry. Such activities are aimed at providing the sufficient maintenance and operational capacity for the purposes of achieving high returns on investments, monitoring the operational patterns, supervising the performance of the installed equipment, as well as maintaining a high quality performance to meet the demands of existing and potential customers (Optimising Asset Management Activities n.d.).
In the case of the PV Module, several engineering asset management activities can be identified. The PV technology requires minimum attendance and maintenance. On the other hand, efficient cleaning and recycling system are the prerequisites for effective engineering management activity (The PV Modules Soiling Issue: Best Solutions 2016). Experts insist that the need to solve the problem of external detriments be of utmost importance.
Various types of substances, such as snow, dirt, dust, often cover the surface of the PV Module, leading to significant soiling losses, i.e. the loss of power. Thus, an efficient and flexible cleaning system must be organized in order to minimize the expenses and reduce potential risks of damaging the equipment. Moreover, the cover surface of the panels loses 3-6 percent of power between rainfall fays, thereby making it a priority to keep the surface clean (The PV Modules Soiling Issue: Best Solutions 2016).
Dust and soil accumulation may also lead to the module being permanently damaged. Thus, the amount of energy generated by the PV module varies depending on the weather conditions (Solar Photovoltaic n.d.). In addition, PV Module recycling is an issue of great significance, as it is intertwined with the question of solar cells decomposition (Fthenakis 2000). Even though this equipment represents a major step towards developing renewable energy sources, there is a pressing need to develop a PB Module recycling system. The equipment discussed should last in good condition for thirty years provided the cleaning system works well (Fthenakis 2000, p. 1). Thus, PV recycling should be addressed as well by developing an efficient system of solar cells, scrap, and module recycling.
All things considered, it is necessary to determine the key engineering asset management decision based on the analysis outlined above. There is a principal need to develop a flexible, smart, and efficient cleaning and maintenance system, as well as a consistent module recycling system. The engineering management of the selected asset should address these questions.
Approach to Life-Cycle Cost Analysis
A life-cycle cost analysis is based on an asset’s life cycle seen as a series of stages, in which the engineering management influences the particular stages of a life cycle. Thus, an efficient approach to life-cycle cost analysis would aim at improving the asset’s overall performance at particular life cycles in their various aspects. According to Hasan, Hossain, and Habib (2015), the asset’s life cycle comprises many subsequent stages, including the process of planning, creating, testing, and deploying a system. Each of these components has a substantial significance to the overall life cycle. The cost-drivers of the life cycle as described by Vikhorev, Greenough, and Brown (2013), constitute the principle reasons for the increasing costs of production, testing, maintenance or deploying a particular system.
The approach that is to address the life-cycle analysis of PV Module should consider several important aspects of the cost-drivers. Firstly, the type of a PV Module and, therefore, the corresponding expenditures, may vary depending on what model of equipment is chosen and what amount of energy it can generate. Thus, taking into consideration the components of a given model and especially its direct applicability, it is possible to develop an approach to the cost analysis of the life cycle.
Firstly, it should be noted that the simplest PV Module model is a model that entail simultaneous generation and consumption (Solar Photovoltaic n.d.). It is the most accessible photovoltaic equipment that is of the lowest cost. Moreover, as the description suggests, it requires a continuous flow of energy so that when energy is generated, it must also be used. Otherwise, the resources are wasted. Secondly, there exists a model that includes a grid tied with a net meter.
The latter generates electricity in a way that supplemental energy returns to the power grid. In this case, the meter will run at a backwards rate, which will reduce the amount of consumed energy (Solar Photovoltaic n.d.). The third possibility is a model off the grid with storage. It is the most complex PV system, as it provides a possibility to store electricity that is not used right away in the non-grid tied framework.
Thus, the entire design of the appropriate PV Module equipment depends on the particular parameters of the venue, as well as on the electricity demands of the building. Therefore, the mentioned cost-drivers can vary according to the particular setting. Moreover, the necessary components of the PV system include a charge controller, diodes, an inverter, and shut-offs (Solar Photovoltaic n.d.).
The system engineering models and life-cycle cost analysis can enhance the decision making regarding actual engineering asset management. The mentioned models provide a foundation for the cost drivers analysis, as well as for the decision making process. By taking into consideration all the presented parameters of the equipment and the applicability of each model, it is possible to improve the decision making, as they provide consistent basis of the necessary costs and expenditures that are correlated with the particular building or a venue. Thus, engineering asset management of the discussed equipment is a process that should involve various aspects of installing the equipment, as well as a correlation with the cost-drivers, and decision-making processes.
Life-Cycle Cost Evaluation within the Life-Cycle Asset Management
Life-cycle cost analysis is a beneficial instrument for designing the suitable PV Module system, as well as for the decision-making process. Such an analysis provides a solid foundation for costs optimization, risk prognosis, timing estimates, as well as determining the appropriate timing for interventions and inspections of the equipment (The value of optimization in asset management 2015). It is necessary to establish the particular benefits that performing life-cycle cost evaluation has on the management of the selected asset.
The PV Module engineering management requires a multifaceted approach that involves professionals of various fields of work: management, information technology, economics, and so on. Such integration poses an organizational difficulty, as it necessitates a high level of coordination and using reliable and valid data. An increased energy demand over the recent years led people to believe it was necessary to develop renewable power sources. The PV Module equipment, as well as solar technology overall, means that humanity makes a step towards preserving the environment. Kamel (2010) emphasizes that one of the advantages of higher energy prices is the capacity of the economy to produce more economic benefits, as compared to the incurred expenses.
Life-cycle cost analysis is a prominent method of predicting the future costs, risks, industry estimates, and other quantitative and qualitative data corpus. Solar technology employed in the case of PV Module, helps provide an additional framework. The determined structures pertain to various aspects of the venue, including electrical wiring, telecommunication system, and other aspects. However, in order to comprehend the role played by life-cycle cost evaluation for the selected asset, it is necessary to correlate the estimates with the life-cycle management.
It should be noted that in the context of engineering management, there are two types of value: capability value and financial value (“What is engineering asset management?” 2010). These values are both based on the degree of their applicability in devising the plan to achieve the goals set out by the company. Capability value is of more importance than financial value to engineers, as it indicates the relevance of the equipment used.
This type of value is usually employed when establishing the physical features of the equipment that might hinder its operational performance. Even though the financial value does diverge from the capability value, together they create a comprehensive picture that incorporates the correlation between the asset’s performance capacity and financial aspects, i.e. the expenses involved in the development of a given project.
In the case of the PV Module, a life-cycle cost analysis reveals that the discussed equipment’s cost usually amounts to a third of the total cost of a Photovoltaic system (Renewable Energy Technologies: Cost Analysis Series 2012). However, as it was demonstrated above by the description of the cost-drivers, the equipment varies according to the needs of the customer, physical building, venue, and site parameters, the required amount of generated power, module efficiency, and so on. Moreover, such essential aspects as site preparation and the installation of the framework incur additional costs. The life-cycle cost estimates indicate additional costs pertaining to the administration and management aspects, as well as to the system of power storage, employed in the off-grid Photovoltaic systems.
Applying the suitable systems engineering models is crucial for the engineering asset management purposes. It is also essential to identify and assure the benefits of applying such models. Model based systems engineering typically include framework architecture, performance analyses, test, as well as a behavioral analysis (Hart 2015). Thus, the life-cycle comprises the appropriate requirements, performance analysis, capability verification, system design, and implementation. Applying the principles of these models helps minimize the risks involved and improve the quality and productivity of operational performance. According to Hart (2015), in order to apply engineering models in an efficient manner, it is crucial to compile a set of the necessary goals and criteria.
Asset Life Cycle Aspect
In their article, Yue, You, and Darling (2014) address a wide range of aspects connected with life cycle costs and PV systems producing electricity directly from solar radiation. As a system is composed of human resources, products, and procedures that give the opportunity to satisfy the identified needs, all these constitutes are addressed. The authors’ approach correlates with the idea expressed by Blanchard and Fabrycky (2011): it is important to make life-cycle considerations a specific aspect of the feasibility analysis process. This section of the paper examines one of the products discussed by the authors, Si-PV modules, that are to become the basis of the new approach towards energy usage and consumption.
First and foremost, Yue, You, and Darling (2014) view the life cycle as a set of stages the combination of which gives the idea of the engineering process Influencing life cycles. As it may be educed from their study, it is probable to enhance the asset performance and keep different processes influencing life cycles via different aspects. The authors’ understanding is close to what some other researchers suggest: a life cycle may be defined as the process of planning, creating, testing, and deploying a system, and each component can be influential (Hasan, Hossain, & Habib 2015). The costs associated with a certain life cycle are called, obviously, “life cycle costs.”
In relation to the product connected with this particular life cycle, it is necessary to point out that it becomes the most important aspect in the context of the system based on PV technologies and promoted by many authors as the technology of the future addressing climate change issues and carbon dioxide emissions. In this context, as the researchers emphasize, they aimed at understanding the advantages and disadvantages associated with these technological innovations and used life cycle assessment to achieve their goal. Consequently, life cycle costs are considered in accordance with the aim.
As it was discovered, the production of PV modules consists of several stages.
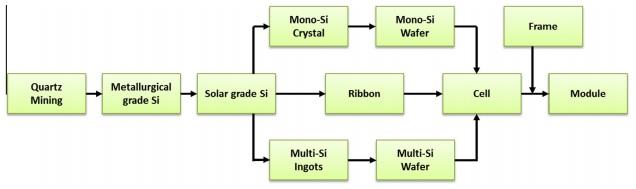
One can see that the authors follow the principle that the relationships between the life cycle cost drivers and the life cycle stages can be described in terms of the time framework. The processes that occur in the course of time are represented by the line. As a rule, the life cycle contains two phases, acquisition and utilization, and several stages, such as conceptual design, detail design and development, production, and so on (Love et al. 2013). In this diagram, the researchers concentrate on the first stage and provide the details of how a PV module is being made.
In this context, it is necessary to refer to the term “cost drivers.” The life cycle cost drivers are recognized as the reason for the costs increase in relation to life cycle (Vikhorev, Greenough, & Brown 2013). In the process of the analysis, it is important to realize that it is the cause of the expenditure that should be examined, not the spending itself. Identifying the cause, one needs to make the search more effective by addressing the spheres of the most influence on overall costs (El-Akruti & Dwight 2013). Cost drivers are used in activity-based cost analysis since organizations, naturally, want to discover the causes before the beginning of their activity.
Taking into account the importance of cost drivers, it is probable to examine the stages associated with the acquisition phase and single out cost drivers, such as the costs of quartz mining, preparing metallurgical grade silicon, manufacturing solar grade silicon, etc. The analysis of the cost drivers is reasonable in the earliest stages since goal setting, assessment of resources, and other significant procedures refer to the beginning of the life cycle. Thus, this approach seems to be relevant.
Further, the authors of the article address the energy payback time and energy return on energy investment. They calculate numerous indexes and argue that the electricity return from PVs depends on the domestic manufacturing scenario: in Europe and China, electricity mix (i.e. the proportion of different types of sources of energy) and energy efficiency differ since China relies on coal to a larger extent in comparison with the European countries and the USA that use renewable energy resources, such as hydropower plants, more (Yue, You, and Darling 2014).
Consequently, the costs are influenced. Europe and America tend to obtain better results. These data demonstrate that PV technologies usage as one of the first-stage components becomes the most important factor that makes an impact on the total costs.
One more asset issue related to the life cycle costs under examination is connected with taxes. The researchers describe the break-even carbon tariff model for Si-PV modules based on the previous calculation as a preferable innovation (Yue, You, and Darling 2014). They suggest a formula that includes several parameters, and one of them, the local manufacturing cost, is influenced by the aspect that is the focus of this section, Si-PV modules as a product. Overall, this aspect is of primary importance in relation to the life cycle cost associated with an asset. It may be stated that the researchers chose the right direction and managed to demonstrate how this aspect works and affects the life cycle costs.
Recommendations
From the perspective of the life cycle aspect discussed above, one can provide several suggestions connected with the cost drivers in the life-cycle analysis for a practical usage in assets administration. While the authors themselves provide some key directions which may bring positive results and help reduce the costs, the list of recommendations may be enlarged. It will be advantageous to follow some of the strategies introduced in other countries, especially those that prove to be effectively using PV modules and demonstrate the improvement in terms of the energy payback time and energy return on energy investment.
- It is significant to draw attention to the technologies enhancement. As the researchers point out, the key characteristics of the Si-PV technologies, such as conversion efficiency, wafer thickness, and material utilization, should be amended (Yue, You, & Darling 2014). To bring technologies to perfection, one may combine the key parameters of the present-day technologies and encourage research in this sphere.
- Following the idea concerning the necessity to carry out research, it is vital to develop the policy that will adequately address the modern demand. The example of countries that developed such programs proves that they generate value: for instance, the USA launched a wide range of programs (PVUSA, Million Solar Roofs program, and so on) (Gambhir, Gross, & Green 2014).
- The third recommendation pertains to the later stages of PV modules usage. As it has been discussed above, China is notable for the high level of coal utilization, and this fact partially explains why the PV modules efficiency is different in Europe and America and, on the other hand, China. Consequently, one of the urgent tasks is to handle the country’s electricity mix and implement technologies based on renewable energy resources.
Conclusion
Photovoltaic Module systems were selected as an asset in the environmental industry. A key engineering asset management decision was identified, as well as the basic activities regarding the PV Modules production, installation, and maintenance. Engineering asset management principles helped gain further insight into the matter. Life-cycle cost analysis helped understand the influence these estimates have on the decision-making process in the engineering asset management.
Reference List
“What is engineering asset management?” 2010, in Amadi-Echendu, JE., Willett, R., Brown, K., Hope, T., Lee, J., Mathew, J., Vyas, N. & Yang, BS (Eds.), Definitions, concepts and scope of engineering asset management (pp. 3-16). Springer, London, pp. 3-16.
Fthenakis, VM 2000. ‘End-of-life management and recycling of PV modules’, Energy Policy, vol. 28, no. 14, pp.1051-1058.
Hart, L 2015, Introduction to model-based system engineering.
Hasan, MM, Hossain, M, & Habib, A 2015, ‘Corporate life cycle and cost of equity capital’, Journal of Contemporary Accounting & Economics, vol. 11, no. 1, pp. 46-60.
Kamel, F 2010, Life-time cost-benefit analysis of solar energy systems in Queensland. Web.
Optimising asset management activities, n.d. Web.
Renewable energy technologies: cost analysis series. 2012. Web.
Solar photovoltaic, n.d. Web.
The PV Modules soiling issue: best solutions 2016.
The value of optimization in asset management 2015.
Vikhorev, K, Greenough, R, & Brown, N 2013, ‘An advanced energy management framework to promote energy awareness’, Journal of Cleaner Production, vol. 43, no. 4, pp. 103-112.