Introduction
In Australia, all types of soils characteristic of the tropical, subequatorial, and subtropical natural belts are represented in a regular sequence. In the region of humid tropical forests in the north, red soils are widespread, changing towards the south by red-brown and brown soils in moist savannas and gray-brown soils in dry savannahs. Red-brown and brown soils containing hummus, some phosphorus, and potassium, are valuable for agricultural use. The principal wheat crops in Australia are located within the zone of red-brown soils. In the outskirts of the Central Plains (for example, in the Murray Basin), where artificial irrigation is developed and many fertilizers are used, grapes, fruit trees, and forage grasses are grown on gray soil. In the surrounding inner desert areas of the semi-desert and especially steppe areas, where there is a grassy, and in some places shrub-tree cover, gray-brown steppe soils are widespread (McKenzie et al., 2004). Their power is insignificant. They contain little humus and phosphorus; therefore, phosphorus fertilizers are required when used even as pastures for sheep and cattle. In this work, thanks to field research, various types of soils are considered. Key features of the studied soils, such as morphology, chemistry, land use issues, and land potential, will be presented below.
Climo-Sequence on Mt. Wellington
Yellow Brown Soils on Solifluction Deposits
Brown soils are typical for mountainous areas. The color of this type of soil has a gradient that gradually lightens from below. The subtropical climate and permanent shelter under evergreen shrubs, forests, and woody vegetation contribute to their formation on highlands and near rivers. One of the main characteristics of these soils is their presence in areas with a non-leaching type of water regime, which is widespread where the average annual precipitation is significantly less than the average annual evaporation. The soil is not soaked to groundwater, and at a certain depth, a dead horizon is formed with a constant moisture content close to the wilting moisture of plants. The middle part of the profile, as shown in Figure 1 from fieldwork, is roughly equal 0.6-0.8 meters, which has consequences for the chemical composition, which will be discussed below.
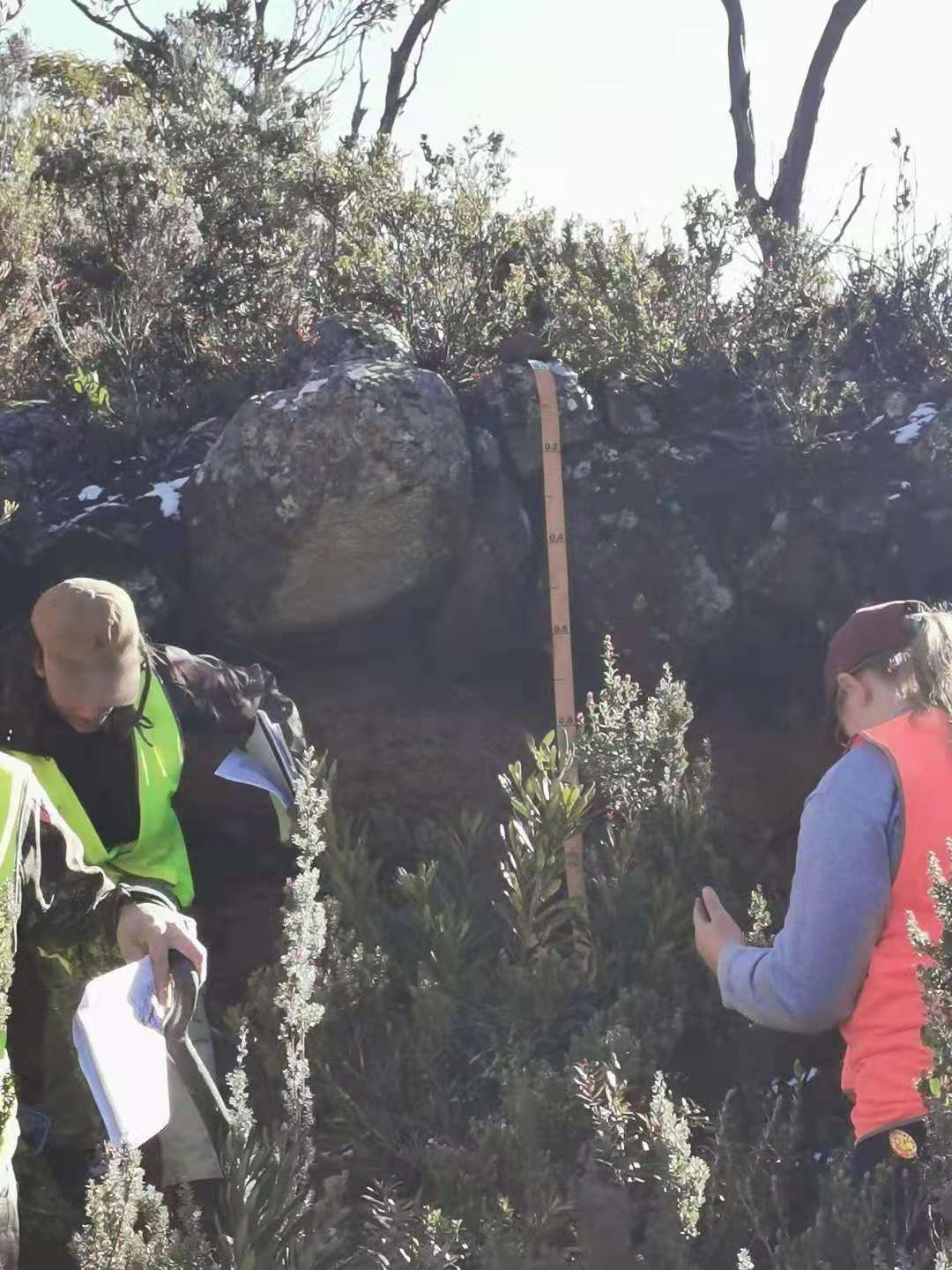
Soliflution deposits are formed due to the thawed and water-saturated soil sliding down the slope along with the waterproof frozen or permafrost layer. In some places, it is not easy to draw the line between diluvial and solifluction deposits, and they are referred to as diluvial-solifluction deposits. A significant part of such soils is highly fertile due to the reasonably deep penetration of humus. Due to the non-leaching type of water regime, the soil is highly saturated with calcium with a high exchange capacity. What is more, the soil’s acidity is neutral; however, when moving down the profile, this indicator, as a rule, increases. The iron base formation serves as an obstacle for the roots on the level of more than thirty cm (Hewitt, Balks, and Lowe, 2021). Finally, the differentiation of the profile for iron and aluminum is quite low.
Traditionally, brown soils are used for viticulture and, to a lesser extent, for fruit growing. Under the conditions of agricultural use, terracing and plantation plowing lead to a substantial change in the soil profile (Ferris and Haigh, 2018). However, the peculiarities of the location of such soils in Australia for humans are purely educational and are not subject to manual processing.
The humus-accumulative subhorizon has a dark gray color, retaining dolerite boulders and small roots of the uppermost subhorizon. The illuvial horizon includes several layers, among which there is the mentioned iron layer. The rest of the sub horizons are characterized by moisture and yellowish-brown color. In this regard, mountain forests located on brown soils have vital water conservation and anti-erosion value. Figure 2 shows a photograph of the profile of this soil. The general graphic of the profile is presented in Appendix A.
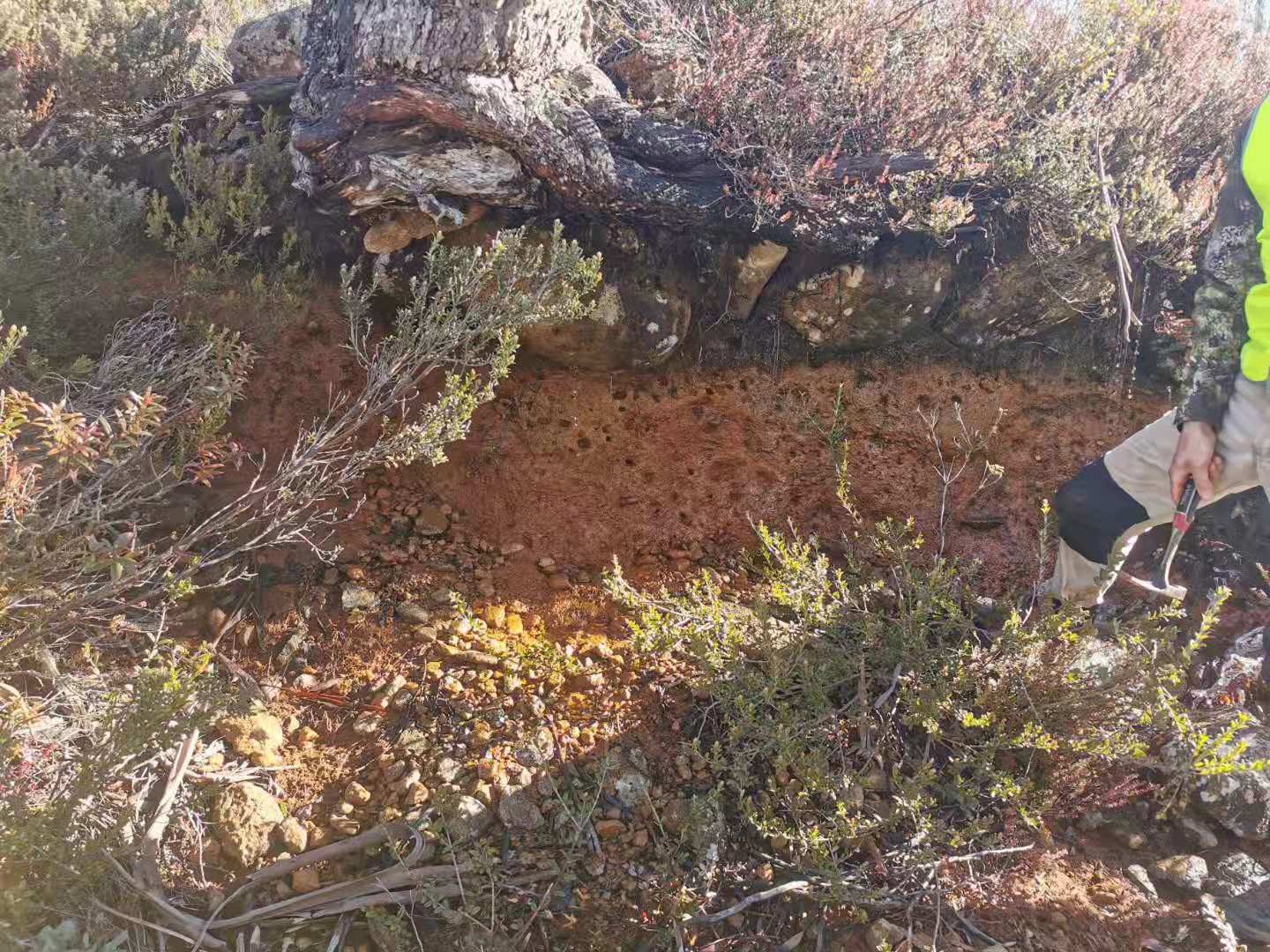
Organogold
Most of the soil profile consists of organic material, which is more often peat, which can vary in botanical composition and a certain degree of decomposition. The thickness of the peat layer ranges from 60 cm to several meters, or the mineral rock goes below the peat layer, which is half a meter – loose gley or dense. The main factor in the formation of peat soils is excess stagnant moisture, which may be the result of atmospheric precipitation or stagnation of groundwater.
Under the peat-litter horizon, a layer with remnants of weakly decomposed mosses is underlain by a diagnostic oligotrophic-peat horizon with a thickness of at least 48 cm, consisting mainly of sphagnum mosses of varying degrees of decomposition, no more than 45-55%. It has a light color and is saturated with water for most of the growing season. The horizon is being replaced by organogenic or mineral rocks. Organogenic rock is peat, the degree of decomposition of which usually increases with depth.
Peat soil is characterized by an acidic reaction (pH value 3.2–4.2), low ash content (2.4–6.0% on the dry matter), and a high level of concentration of macronutrients in the upper part of the soil layer, which exceeds 30% of the horizon mass. The absorption capacity is 80–90 mg-eq / 100 g. The gross content of CaO, K2O ranges from hundredths to tenths of a percent. High nitrogen content with insignificant participation of mobile is one of the main characteristics of this type of soil, mainly ammonium forms. The biological activity is deficient. The profile of oligotrophic peat soil is usually water-saturated (Butterly, Sale, and Tang, 2017). Water permeability decreases with depth, especially in thick peat deposits. The moisture capacity of soils reaches 700-1500% moisture on dry matter, confirmed by the profile photo in Figure 3.
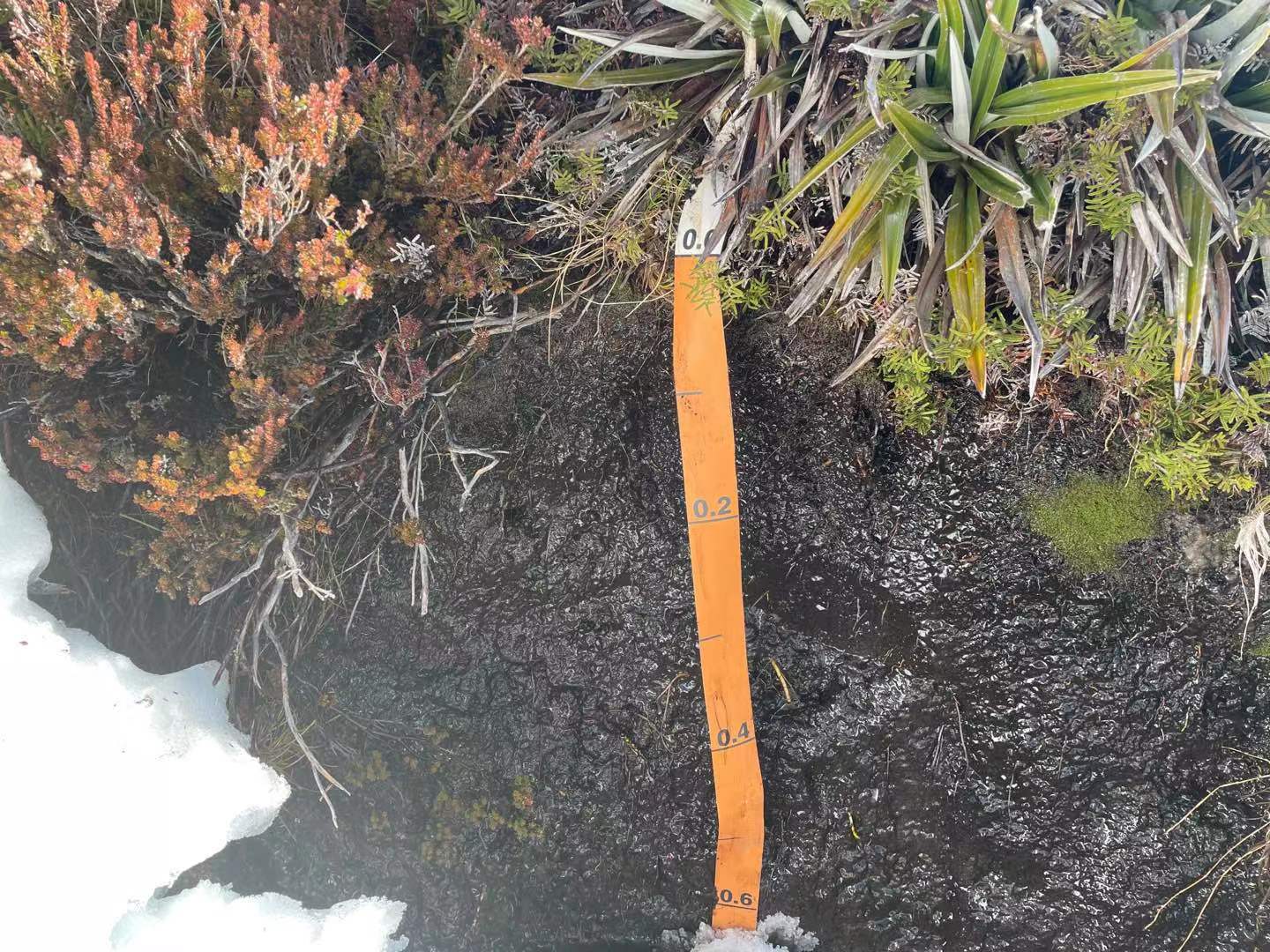
Peat soils are not suitable for any use due to their water regime, physical and chemical properties. Given their specific location in the mountainous region, a high fire hazard in dry seasons, and high phosphorus content, the land potential of these soils is minimal. The material and its structure are shown in the photograph in Figure 4.
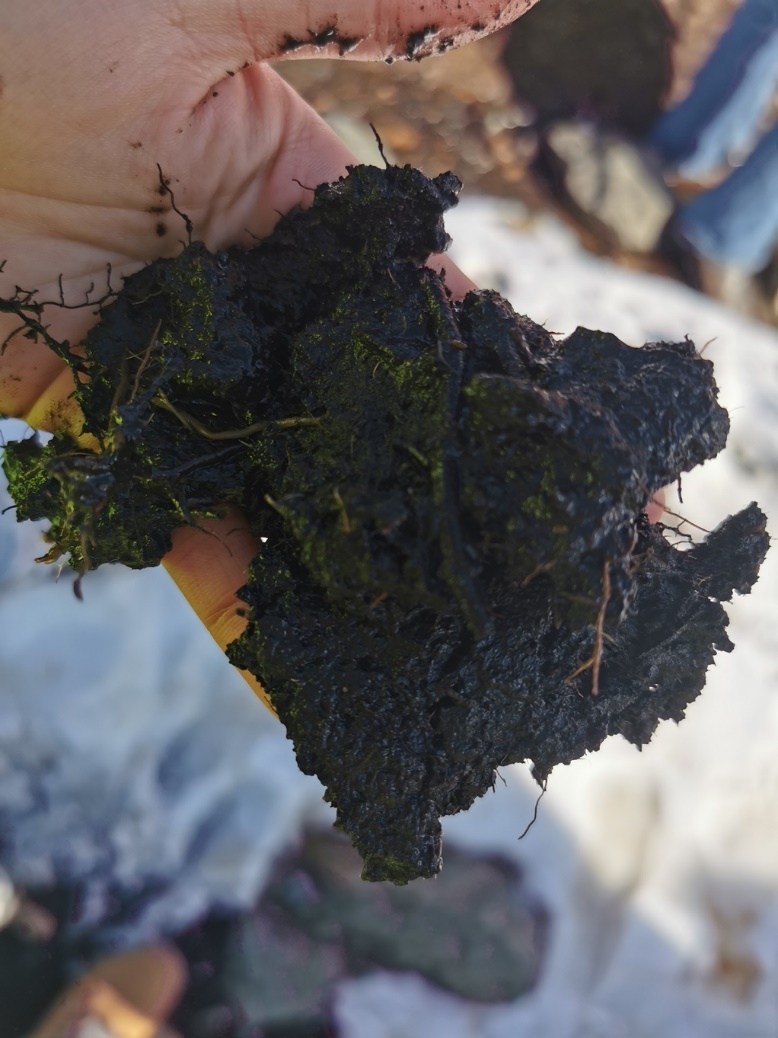
Mt. Rumney Soils
This section deals with the red-brown soil type. The profile consists of horizons sharply different in morphology, composition, and properties. The upper humus horizon, as a rule, has a light granulometric composition; in field conditions, it is defined as sandy or sandy loam. Its bulk comprises pseudo-sand – fine clay and sand particles, very firmly cemented into micro aggregates by dehydrated iron oxides. This material behaves like natural sand in terms of physical properties (filtration, aeration, moisture capacity).
Below lies a dense, almost structureless clayey horizon, illuvial enriched with clayey material. It is uniformly impregnated with iron oxides, which cause micro-aggregation of the material. Further, there is a horizon of ferruginous nodules, and even lower – a horizon of ferruginous-calcareous nodules – kankar (Zhao et al., 2021). The combined accumulation of iron oxides and lime in concretion form is a unique phenomenon that occurs only in red-brown savanna soils.
The high iron content of the profile gives the soil a bright red-orange or brick-red color, which is also specific for these soils. In the absence of a protective vegetation cover, the light surface horizon is quickly destroyed by torrential rains and is demolished, and a dense clay-ferruginous mass appears on the surface, cemented under the influence of direct solar irradiation. As a result of the excessive use of these landscapes in cattle breeding or agriculture, red-brown savanna soils have been eroded over vast areas and turned into badlands with a surface covered with a cover of ferruginous and carbonate nodules.
To a large extent, the red-brown savanna soils are currently used as pastures, but to some extent, agriculture is also spread on them, especially for growing peanuts, cotton, and corn. With low farming technology and the absence of anti-erosion measures typical for countries with a wide distribution of these soils, erosion processes and the transition of productive ecosystems to badlands are highly developed. The red-brown profile in Mt. Rumney is shown in Figure 5. The general graphic of the profile is presented in Appendix A.
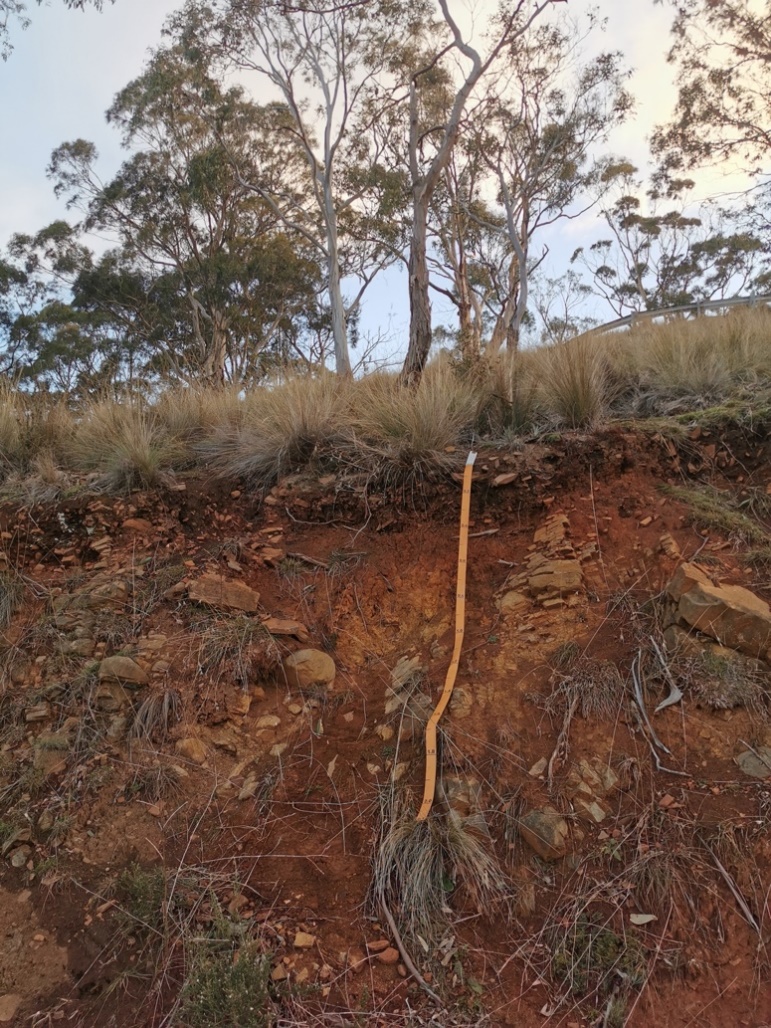
Brown Chromosols/Sodosols
The profile of these soils is often formed on windy sandstones or plateaus. The soil surface consists of sandy loam, olive and brown, and neutral acidity, which increases slightly when moving down. In the depths of the soil, there is a solid gray clay with a still slightly acidic pH. The physical properties of these soils are usually good enough for agricultural use but may become too hard after long cultivation (Marchuk, S., and Marchuk, A., 2018). In addition, there is the potential for seasonal waterlogging due to poor internal drainage and fairly good root and water movement.
Nevertheless, the agricultural potential of the soil in the soils of the chromosol and sodosal species is relatively low due to the different chemical properties. The high sodium in soda salt contributes to soil erosion and very low permeability. Chromosol can be subject to acidification and leaching of nutrients. The acidity increases as it approaches the parent rock C. The general graphic of the profile is presented in Appendix A.Figure 6 shows the soil profile from fieldwork.
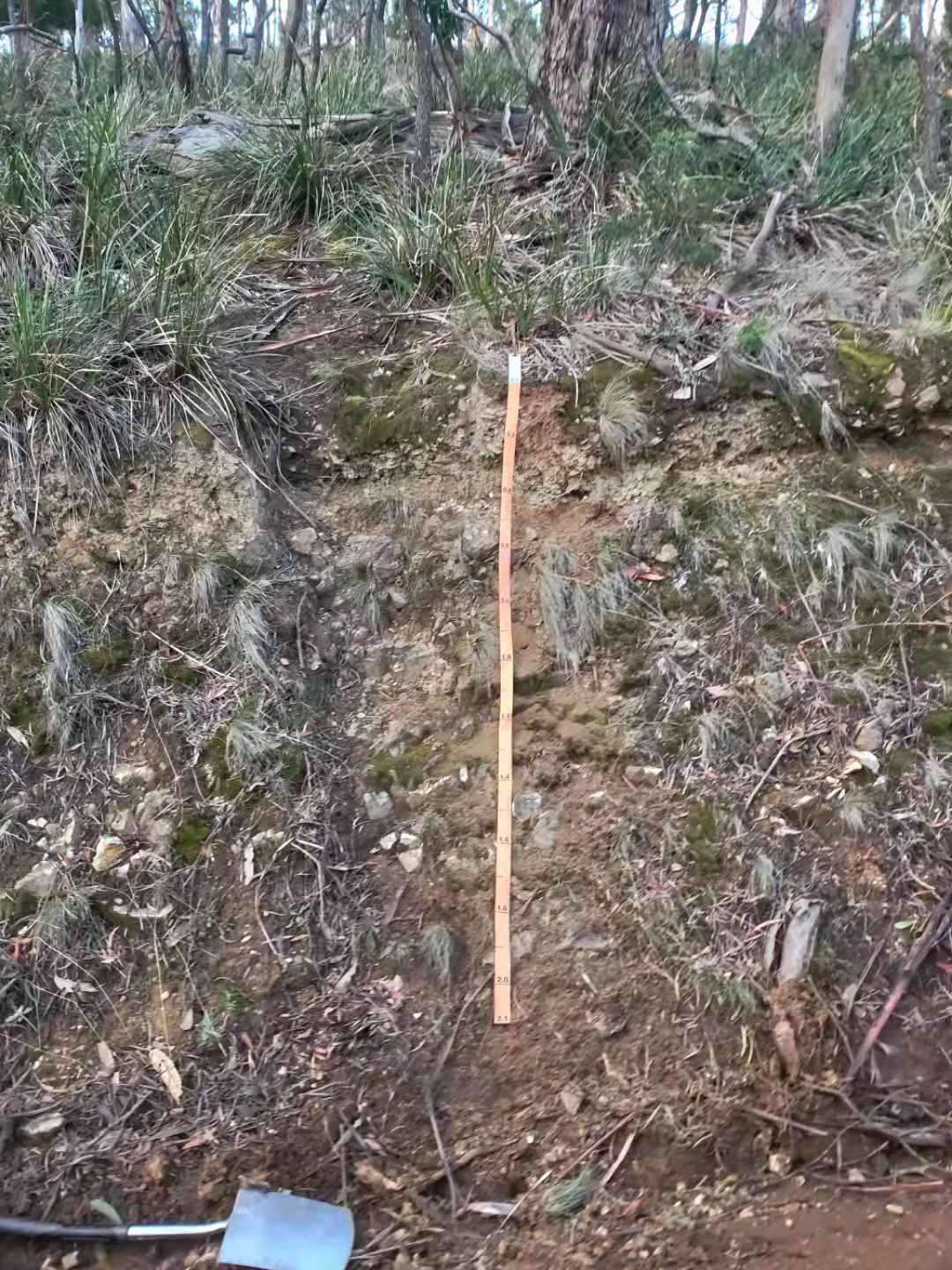
Podosol
Podosols are formed in humid regions’ lowland and mountainous areas, mainly from the forest-tundra to the southern taiga, on sediments of a light granulometric composition (sandy-sandy loam and stony-fine earth), which provide good internal drainage of the soil layer. The vegetation is represented by pine, spruce pine, and larch-pine forests.
Illuvial-ferruginous podzols are formed mainly on mono mineral sands or eluvial-diluvium of silicic igneous rocks and tend to less humid facies and xeromorphic positions in the relief. Illuvial-humus podzols are formed on the weathering products of massive crystalline rocks, relatively rich in minerals unstable to weathering and polymineral sands. They are typical for more humid regions and less drained parts of slopes.
Podosols are characterized by a differentiated profile, consisting of a slight peat litter O, 3–8 cm thick; a thin organic-mineral horizon AO, whitish, clarified due to the removal of coloring iron compounds, and humus of the podzolic horizon E with a thickness of 2 to 20-30 cm and the alpha-humus horizon Bhf of brown or ocher tones, formed as a result of the illuvial accumulation of aluminum-ferruginous-humus complex compounds gradually passing into the parent rock C (Hughes et al., 2017). Poverty in nutrients, acid reaction, and low water-retention capacity complicate the agricultural development of podzols. When using soils for arable land, liming, the introduction of increased doses of organic and mineral fertilizers, a unique farming system, different from loamy podzolic soils, are necessary. The main crops are potatoes, winter rye, and many more. It is most expedient to use podzols in forestry.
Podosols are characterized by an acidic and very acidic reaction of the entire profile, low absorption capacity and degree of saturation with bases, accumulative or eluvial-illuvial distribution of humus of fulvate, sometimes humate-fulvate composition, eluvial-illuvial differentiation of silicate and non-silicate forms of iron and aluminum oxides. A clear profile eluvial-illuvial redistribution of sesquioxides occurs against the background of general desilication of the profile and its enrichment with sesquioxides compared to the parent rock. The distribution of the silt fraction is often uniform or accumulative, but it can also be eluvial-illuvial. C Heterogeneous in microstructure: microzones with compact packing of mineral material with a low content of finely dispersed matter and microzones enriched with it are distinguished. In the material of the sand-plasma microstructure, a high optical orientation of the plasma and clay curtain on the grains of minerals is noted. The signs of mineral weathering are weakly expressed – thin ferruginous films along cleavage cracks of ferrous minerals (pyroxenes, amphiboles, biotite). A photograph from the field works is shown in Figure 7. The general graphic of the profile is presented in Appendix A.
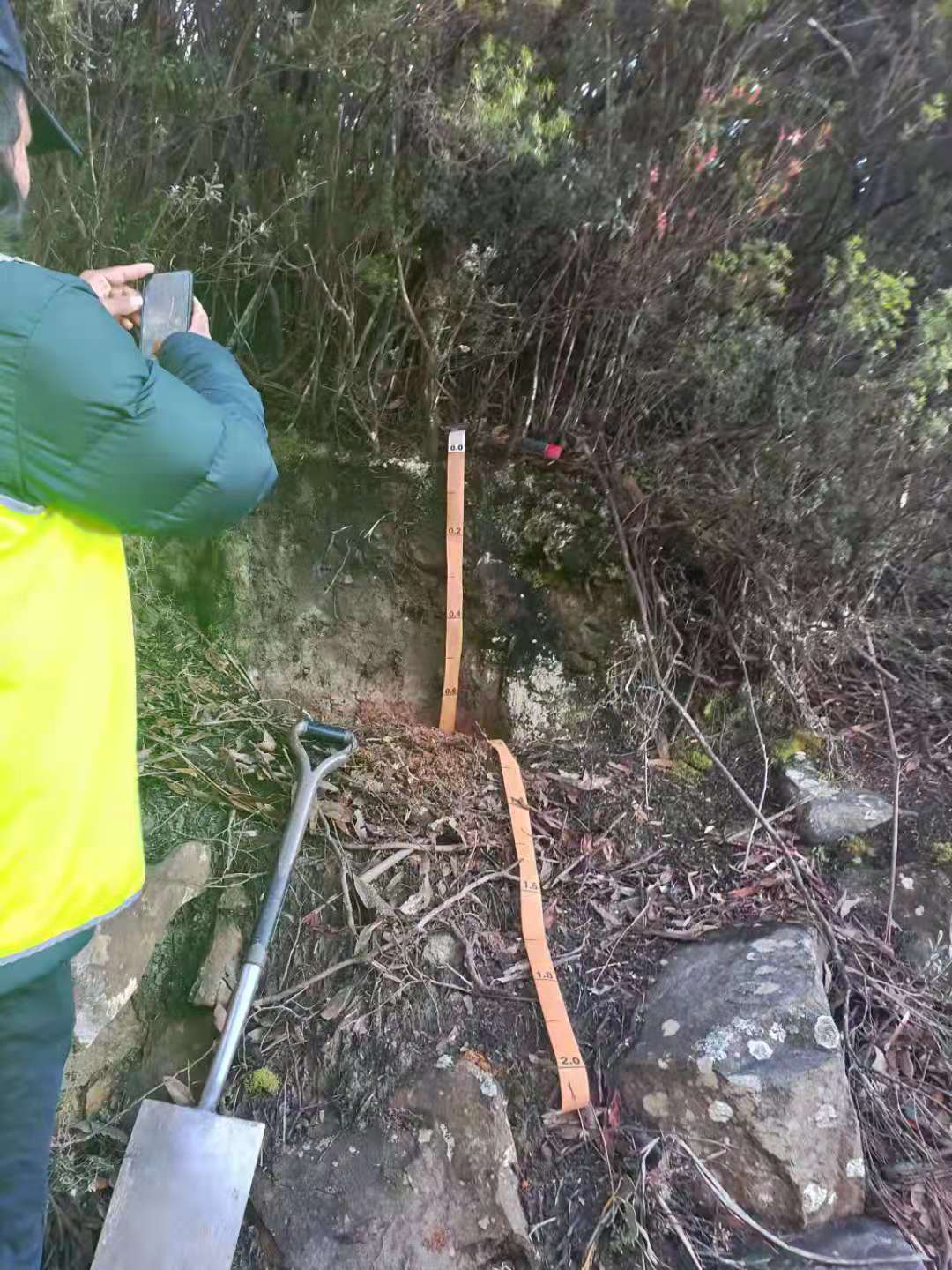
Kurosol/Kandosol
Kandosol does not have a solid textural contrast between horizons A and B. They have a relatively solid and massive horizon B with no carbonate substances. These soils are found in low drainage areas, usually yellow or gray, with rainfall ranging from 400 mm to 1300 mm. At the same time, in well-drained areas (brown and red kandosol) with rainfall from 200 mm to 1500 mm. Generally, kandosol has low to moderate agricultural potential with moderate chemical fertility and water retention.
Kurosol is also not very suitable for agricultural activities due to its high sodium content and acidity values. Low water retention capacity and low chemical fertility only confirm these facts. Nevertheless, in some regions, pastures and orchards are created on such soils, but the potential of such land, in any case, remains low. The profile is notably dry; the color varies from gray to yellow-brown. A photograph from fieldwork is shown in Figure 8. The general graphic of the profile is presented in Appendix A.
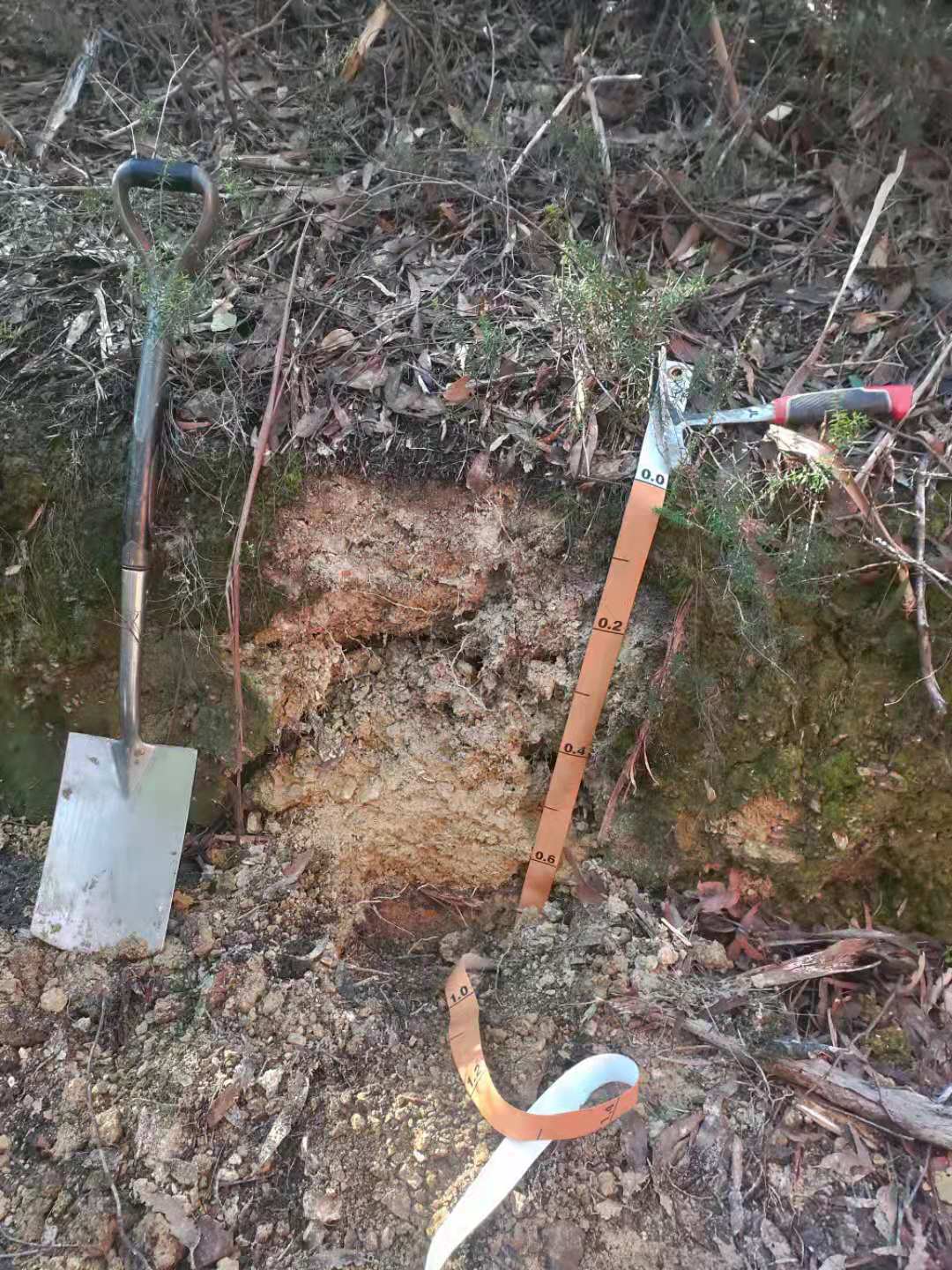
Soil Formation on a Range of Different Soil Parent Materials
Brown Dermosols
This type of soil is characterized by a gradual transition of the profile structure, in contrast to the kurosol mentioned above or sodosol. The primary color of this type of soil is brown with yellowish and reddish tints. The silty loam in the upper profile is characterized by highly high acidity (from 4.8 and above), as well as the multifaceted clays below. Weathered sedimentary rock C is found at a depth of one meter and below. The low content of free iron in the middle of the profile is a chemical characteristic of this type of soil (Amoah-Antwi et al., 2020). This type of soil is located, as a rule, in areas with little drainage, but with abundant rainfall, or vice versa – in regions with good drainage and not too heavy rainfall. Excellent structure, chemical fertility and high water retention rates make the land potentially suitable for agricultural use.
Brown colors and tones are the main colors of this soil. Under the thin litter lies a lumpy-granular humus-accumulating horizon A, brown with a gray tint. It is followed by a brown, and sometimes brown and compacted horizon Bm. Then it gradually merges into the lighter carbonate rock Cca. Effervescence from hydrochloric acid usually begins in the metamorphic layer or transition to the parent rock horizon, and carbonate accrues in the soil.
The soils are characterized by a high humus content of the upper horizons (under natural vegetation – up to 5–7%) and immersion of humic substances (0.9–1.1% humus at a depth of approximately 100 cm). The composition of humus is fulvate-humate (Cg / Cfk about 1), humic acids associated with calcium prevail. Claying of the entire soil layer, especially the middle part, is associated with the accumulation of silt due to intrasoil weathering. Along with the high capacity of cation exchange and the complete or almost complete saturation of the absorbing complex with bases, brown soils are characterized by the accumulation of non-silicate (mobile and crystallized) iron, the maximum of which is confined to the horizon of the most extraordinary clay formation (Murphy, Greene and Harms, 2017). The textural differentiation of the profile is quite characteristic in the absence of differentiation in terms of the gross chemical composition. Soils studied in the fields are presented in Figures 9 and 10. The general graphic of the profile is presented in Appendix A.
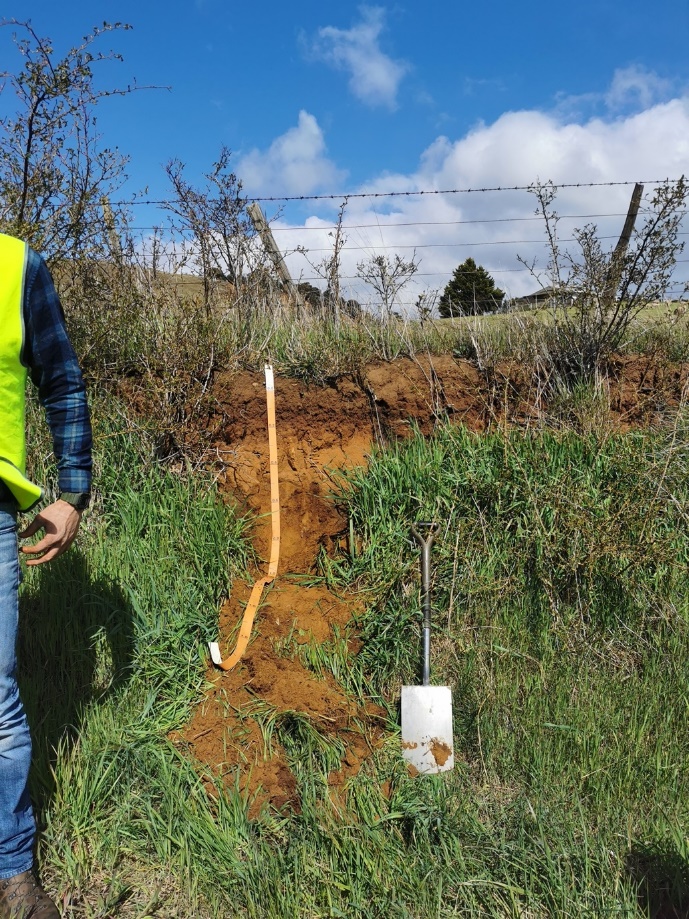
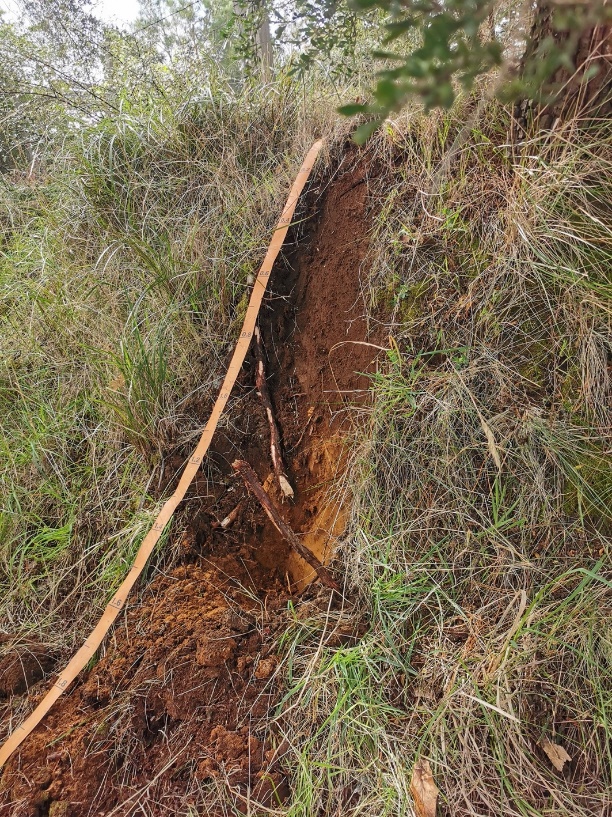
Black Vertosols
Black vertosols are confined to the northern part of the steppe zone with a semiarid climate. They are formed on loesses and loess-like loams or eluvial-diluvian of bedrocks, mainly of clay-loamy composition, under the forb-sod grass northern (true) steppes. In actual steppes, in comparison with meadow ones, the amount of forbs and rhizomatous grasses decreases in the composition of vegetation, and the number of roots relatively increases in comparison with the aboveground part of plants. The water regime is non-flush; through, or deep wetting in these soils is very rare.
Black vertosols have a dark gray or black color humus horizon with a distinct granular or granular-lumpy structure. The thickness of the humus-stained strata (A + ABca) is usually 40–80 cm. Effervescence from HCl has been noted the A (ca) horizon or in the part of ABca. Carbonate precipitates appear somewhat below the effervescence line in the form of rare pseudomycelium or dark impregnating spots; their maximum in the form of a white-eye is concentrated in the Bca horizon. At a depth of 300–500 cm, precipitates of gypsum and readily soluble salts can be observed.
Black vertosols have a great level of natural fertility; their plowing is high too (50–75%). The main direction of agriculture on these soils is a grain (spring and winter wheat, corn). Large areas are occupied by industrial crops (sugar beets, tobacco, sunflower). Meat and dairy cattle breeding is well developed. In arable soils, water and wind erosion, dehumidification, and over consolidation are widespread (Mansergh and Heinze, 2019). In agricultural use, measures for the accumulation and conservation of moisture in the soil and protection of soil from erosion are of great importance. To obtain high yields, the combined application of mineral and organic fertilizers is practical.
Black vertosols are similar in properties to typical ones, but the process of humus accumulation is weakened, the humus content rapidly decreases with depth. The humus horizon, with a 5–8% humus content with a heavy granulometric composition and 4–5% with a light one, is characterized by an excellent macro and microstructure. The composition of humus is humate-calcium (Cg / Cfk about 2). A neutral reaction characterizes the soils, a high absorption capacity (40–55 mmol (eq.) / 100 g of soil), and an absorbing complex completely saturated with bases. The distribution along the profile of the silt fraction, sesquioxides, and silica is uniform (Malone and Searle, 2020). A well-defined water-resistant structure provides a favorable water-air regime.
Layer A is characterized by a dark gray color, spongy micro formation, multi-order organization of aggregates, a massive amount of excrement of soil mesofauna (earthworms, enchitreids), high inter-and intra-aggregate porosity, the predominance of coagulated dark-colored humus (humus of the “mull” type) and high amount of small highly decomposed plant tissues, clay-humus plasma, isotropic (Arnold et al., 2020). ABca is heterogeneous in microstructure; there are zones and aggregates with different contents of finely dispersed dark humus, carbonate-clay plasma with crystalline optical orientation prevails, in some zones plasma of clay-humus composition, porous material with a large number of biogenic pores, fine-grained calcite appears in some pores.
Vsa is distinguished by clay-carbonate plasma with crystalline optical orientation and a wide variety of carbonate new formations – scattered micro-grained calcite prevails in the groundmass, its increased concentrations around and inside the pores, fine-grained new formations are found in the pores of the channels, the acicular form of calcite forms cutans in biogenic pores-channels. Boca is a highly porous micro aggregate material with a clay-carbonate crystalline plasma, there are rounded carbonate-clay aggregates-ooids with a concentric optical orientation of clay, and fine-grained calcite crystals are found in the biogenic pores of the channels. The results of fieldwork are shown in Figure 11. The general graphic of the profile is presented in Appendix A.
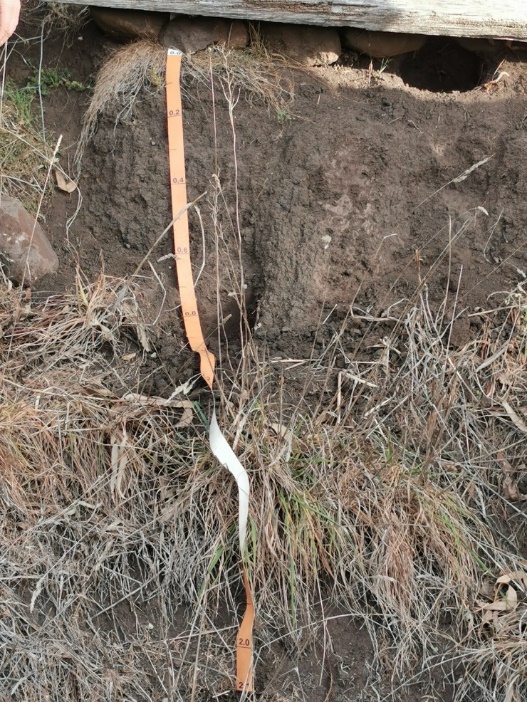
Soil Formation Through Time on Alluvium
Alluvial Soils of the Modern Flood Plain
Alluvial soils are synlithogenic soils; soil formation occurs in them simultaneously with the formation of parent rocks: with the accumulation of fresh mineral material. Its constant supply to the soil surface: leads to constant rejuvenation of the substrate; causes the upward growth of the soil profile and the burial of previously formed horizons.
As the material of different granulometric compositions tends to accumulate, the formation of a layer of varying degrees of occurrence also occurs, which is the main reason for soil formation. For this reason, the soils of the department do not have a typical diagnostic horizon. Alluvial soils are formed under the conditions of the floodplain regime – the regular deposition of layers of the fresh river or lacustrine alluvium of different granulometric compositions on the surface of the floodplain. The thickness of the layers varies from a few millimeters to 10-20 cm. Most alluvial soils are characterized by a profile consisting of an organogenic or humus horizon, gradually giving way to a layered stratum (with buried humus horizons), or combined with a gley, quasi-gley, or fused horizon, as well as horizons hydrogenic accumulation of iron and carbonates.
Alluvial meadow soils in their natural state have a well-defined sod, a powerful humus horizon of dark gray or gray color is distinguished by a distinct granular or lumpy-granular structure and an abundance of earthworm coprolites. On the lower layers of a given soil, traces of gleying are often observed, and sometimes signs of gathering of iron compounds and carbonates – in the floodplains of southern rivers, the accumulation of soluble salts is possible.
The provision of alluvial soils with moisture and plant nutrients due to regular floodwaters and alluvium deposits creates favorable conditions for cultivating crops that are demanding on fertility – vegetables, fruits and berries, sugar beets. These soils are also used in meadow growing, growing forage crops, and under pastures.
The environmental (especially water protection) role of natural soils and biocenoses of river valleys is very high; therefore, the involvement of alluvial soils in agricultural production requires caution. Alluvial soils differ markedly in analytical characteristics, forming in different bioclimatic and geomorphological conditions on the alluvium of different granulometric and mineralogical compositions under the influence of ground and surface waters of different mineralization. Their reaction varies widely; they are acidic, saturated (neutral), carbonate, and saline (saline). The structure and content of organic substances, cation exchange capacity and the degree of saturation with bases can take on completely different values (Bowd et al., 2019). A photograph from fieldwork is shown in Figure 12. The general graphic of the profile is presented in Appendix A.
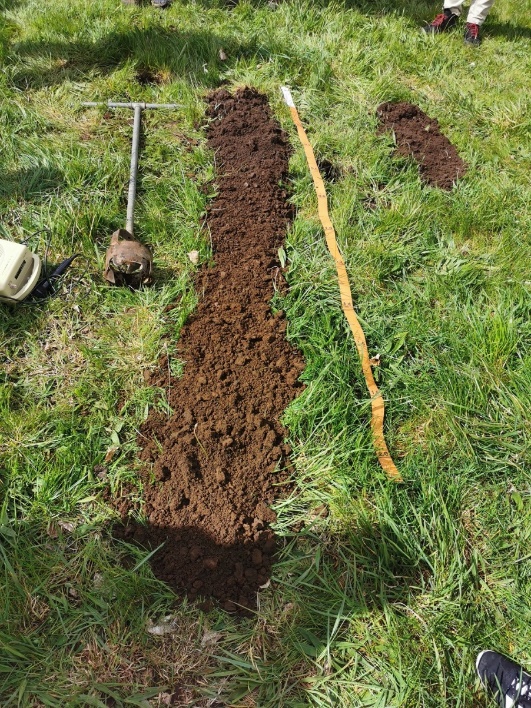
Brown Sodosols on Lower River Terraces
Brown sodosols on lower river terraces – soils formed on alluvial deposits of floodplains of large rivers. They differ by high biogenicity, layering and the presence of low humus horizons. The processes that form the soils in their final form serve as the basis for dividing this species into specific types: for example, soddy ones – are formed under grass-forb meadows and light forests on the banks of channels and ridges. They differ in light composition, unstable water regime, determined by precipitation and floods. The meadow is formed, as a rule, near the center of the floodplain itself, using herbaceous vegetation as a reference point. For this, however, special conditions of soil moistening are distinguished, contributing to the accumulation of humus and gleying of the lower horizons. Bogs are formed in the near-terrace part of the floodplain; they are confined to harmful forms of relief – depressions. They are characterized by peat content, siltation, and the forest zone’s floodplains – by intense gleying. Limestone and salinization are formed in the floodplains of rivers and different zones in bogs.
Floodplain meadow-boggy soils have a particular morphological structure. The surface layer is a dense dark gray-brown sod layer, the entire thickness of which is penetrated by the roots of herbaceous plants. Beneath it, there is a humus horizon of a granular structure with red and brown patches. Both upper horizons provide soil fertility and the advantageous chemical properties of the land for successful farming. Two transition layers follow these horizons. One of them is granular, containing ferruginous inclusions; the second is vicious, unstable, gradually turning into a state of gleying, due to which its fertility is significantly reduced. Dandy, layered alluvium deposits are most often located in lower layers, and an aquifer is located above the parenting horizon.
The use of the floodplain part of rivers in agriculture is sometimes complicated by significant differences in the relief, not only by the composition of the soils. Annual floods and floods in spring inevitably flood low-lying areas that need to be drained constantly, and they are simply difficult to handle. Despite the high potential and fertility, especially the meadow subtype, these lands are mainly left for hayfields, and only a tiny part is allowed to grow moisture-resistant cereals and fodder vegetables (Murphy et al., 2018). A photograph from fieldwork is shown in Figure 13. The general graphic of the profile is presented in Appendix A.
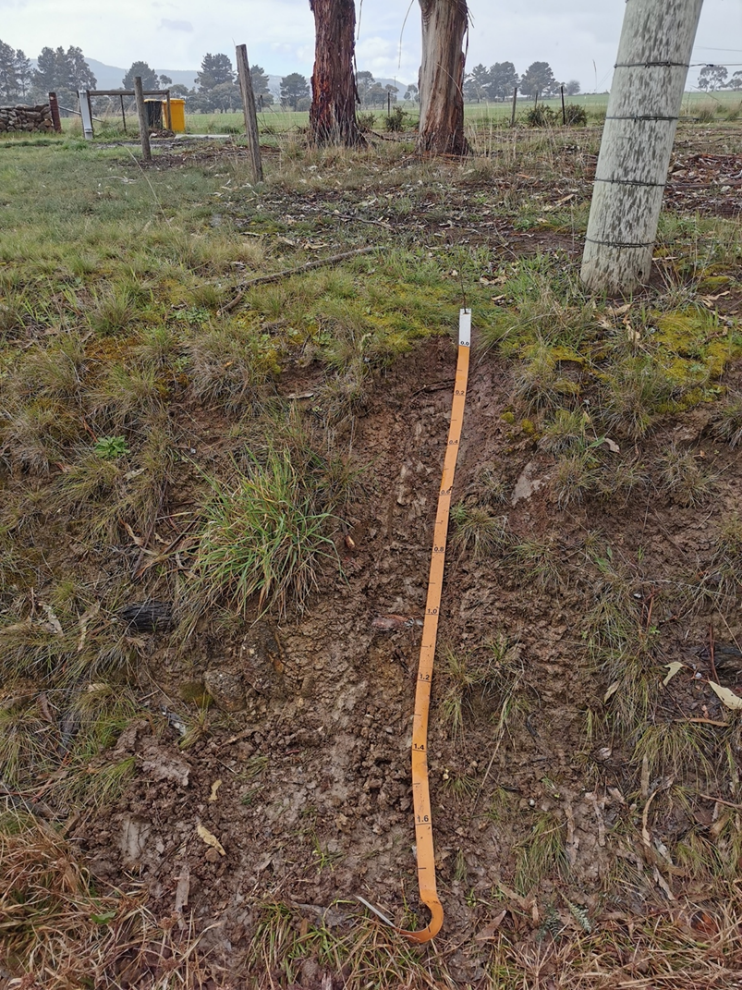
Brown Kurosols (Lateritic) of Higher River Terraces
The profile includes a gray-humus (soddy) horizon of gray or brownish-gray color, lumpy, often with poorly diagnosed bedding; the sod is usually well developed. Traces of soil fauna activity are visible, while the horizon is 20–30 cm thick, rarely more. Soils can have any particle size distribution. At the same time, due to the excellent structure of the gray-humus horizon, they are distinguished by good water permeability and aeration, the predominance of descending moisture currents. The content of humate-fulvate humus is 3–6%, sometimes up to 10%. The reaction of the medium is acidic or slightly acidic (pH <6), the saturation of the absorbing complex with bases is 60–80%. They form on relatively formal relief elements of the central floodplain under grass meadows and floodplain forests under conditions of short-term flooding with open waters. Such soils of the soils of floodplains of small rivers are used, as a rule, for hayfields and pastures.
In most cases, vegetables are grown on the floodplains of large rivers. Depending on the regime of the river, there may be a need for hydro-technical reclamation – the construction of dams that protect the fields from solid floods. Very often, brown kurosols soils are irrigated without significant adverse effects (Beveridge, Williams, and Adkins, 2020). Due to their high sensibility on soils of light granulometric composition, the anthropogenic impact should be limited as much as possible. A photograph from fieldwork is shown in Figure 14. The general graphic of the profile is presented in Appendix A.
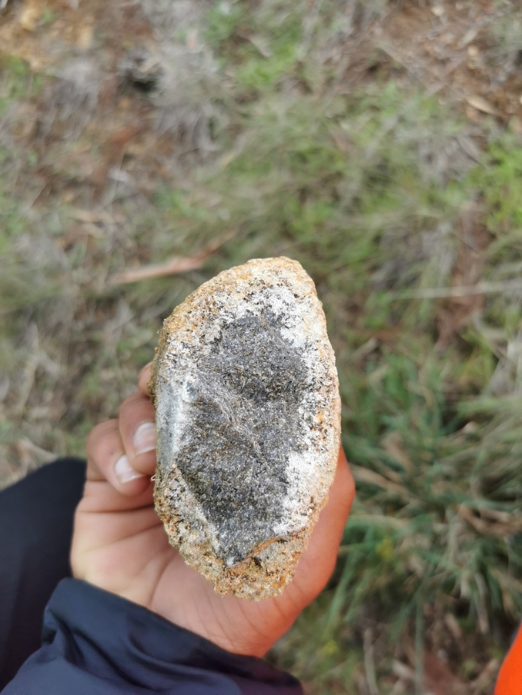
Conclusion
Australia is a continent of relict soils and stark soil contrasts, so both highly moist and arid soils are widespread on its territory. Arid zones and arid sands cover about a third of Australia’s land area. The texture of many Australian soils is characterized by gravel, nodules, and fine sand. Soils with kaolinite clayey horizons are often found, associated with intense weathering in the previous tropical climate. Clay montmorillonite soils are formed in a dry tropical climate.
The main soil-forming processes are ferritic, the formation of swelling clays, the formation of a textured clay horizon B, insignificant peat accumulation, secondary salinization during desertification, and salinization of undrained closed-drainage areas. Salinity and salinity are developed locally in depressions, in oceanic regions. These fieldworks made it possible to study the soils above life, get acquainted with their structure by touch, and evaluate the color and profile depth in places where the terrain allowed it to be done. As a result, as a result of this work, knowledge and experience in working with soils were obtained, which will be helpful for further research.
Appendix A
Soils Profiles
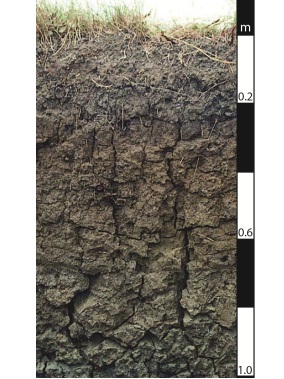
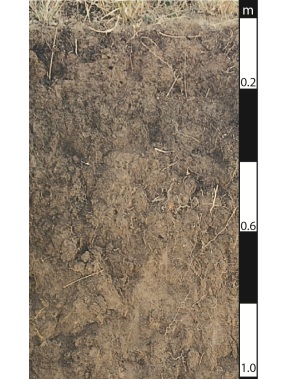
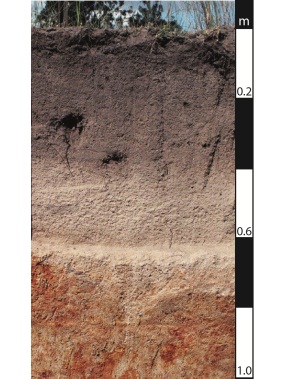
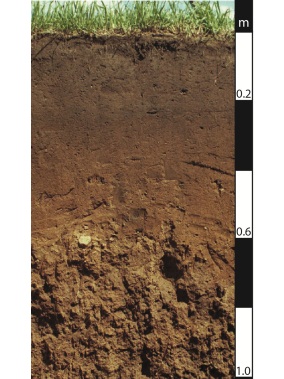
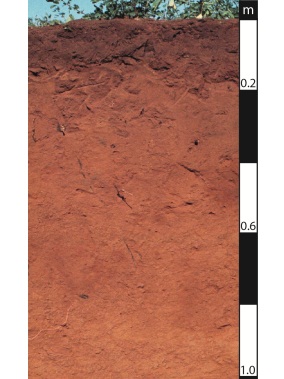
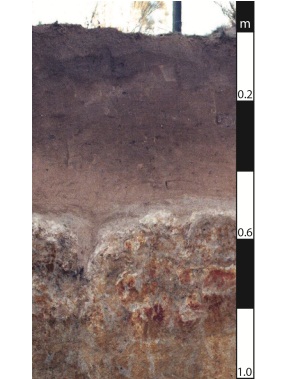
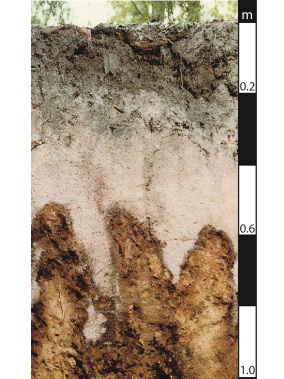
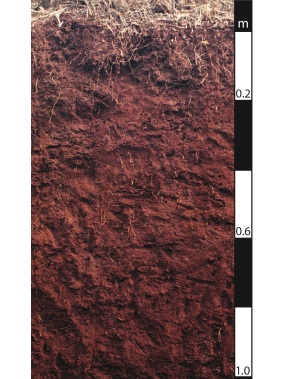
Reference List
Amoah-Antwi, C., et al. (2020) “Restoration of soil quality using biochar and brown coal waste: A review”, Science of the Total Environment, 722, pp. 137852 – 137858.
Arnold, S., et al. (2020) “Event‐based deep drainage and percolation dynamics in Vertosols and Chromosols”, Hydrological Processes, 34(2), pp. 370-386.
Beveridge, F. C., Williams, A., and Adkins, S. W. (2020) “Seed enhancement technologies to improve germination and emergence of Australian native Poaceae”, Seed Science Research, 30(4), pp. 293-303.
Bowd, E. J., et al. (2019) “Long-term impacts of wildfire and logging on forest soils”, Nature Geoscience, 12(2), pp. 113-118.
Butterly, C., Sale, P., and Tang, C. (2017) “Effectiveness of common brown manures and Ca (NO3) 2 in ameliorating acid soils” In “Doing More with Less”, Proceedings of the 18th Australian Agronomy Conference 2017, Ballarat, Victoria, Australia, 24-28 September 2017 (pp. 1-4). Australian Society of Agronomy Inc.
Common Soil Types. (n.d.)
Ferris, I. G., and Haigh, B. M. (2018) “Herbicide persistence and movement in Australian soils: Implications for agriculture”, In Pesticide Interactions in Crop Production (pp. 133-160). CRC Press.
Hewitt, A. E., Balks, M. R., and Lowe, D. J. (2021). Anthropic Soils. In The Soils of Aotearoa (pp. 41-55). Springer, Cham.
Hughes, P., et al. (2017) “Comparisons between USDA Soil Taxonomy and the Australian Soil Classification System I: Data harmonization, calculation of taxonomic distance and inter-taxa variation”, Geoderma, 307, pp. 198-209.
Malone, B., and Searle, R. (2020) “Improvements to the Australian national soil thickness map using an integrated data mining approach”, Geoderma, 377, pp. 114579.
Mansergh, I., and Heinze, D. (2019) “Bogong Moths’ Agrotis infusa’, soil fertility and food chains in the Australian alpine region, and observations concerning the recent population crash of this iconic species” The Victorian Naturalist, 136(6), pp. 192-202.
Marchuk, S., and Marchuk, A. (2018) “Effect of applied potassium concentration on clay dispersion, hydraulic conductivity, pore structure and mineralogy of two contrasting Australian soils”, Soil and Tillage Research, 182, pp. 35-44.
McKenzie, N., et al. (2004). Australian soils and landscapes: an illustrated compendium. Clayton: CSIRO publishing.
Murphy, B., Greene, R., & Harms, B. (2017) “The nature and classification of Australian soils affected by sodium” In EGU General Assembly Conference Abstracts (p. 12294).
Murphy, D. V., et al. (2018) “Soil quality indicators in Western Australian farming systems”, Farming Systems Updates, 109.
Zhao, D., et al. (2021) “Predicting soil physical and chemical properties using vis-NIR in Australian cotton areas”, Catena, 196, pp. 104938 – 104939.