Introduction
Tensile testing is vital a test synonymous with material scientists who subject a material sample to a controlled tension force until the yield point where the material fails. The essence of performing this test is that it aids in quality control, selection of a material meant for a specific application, and gives a glimpse of how a material would behave under varied forces (Simons1970). Fundamentally, the parameters obtained directly as a result of this test are maximum elongation, definitive tensile strength, and reduced cross-section area. In a nutshell, from the aforementioned parameters, one is in a better position to determine: Poisons ratio, Young’s modulus, strain-hardening, and yield strength characteristics (Alexander and Street 1976).
Background literature
Also known as material engineering, material science is a multidisciplinary field that incorporates the properties of matter in scientific cum engineering aspects. To this end, it links the microscopic properties of a material at its molecular level to its larger macroscopic properties (Higgins 1971). In synopsis, it encompasses both chemistry and applied physics. Perhaps, one may wonder the significance of this knowledge in an aeronautical field, but it is central with regards to forensic engineering or failure analysis in case of an accident. Fundamentally, the physical properties exhibited by material are a manifestation of the chemical composition, and the ensuing processes (thermal) that eventuates in the formation of a final product. Critically, the ensuing thermal processes determine a sample’s micro-structure and hence its physical properties (Hashemi 2001).
Material science and alloys are two inseparable entities. Basically, in “the world today, of all the metallic alloys in use none surpass iron’s (cast iron, steel and stainless steel) with respect to preference and commercial value” (Day 1988). Whatever determines the characteristics of this alloy is the degree of carbon, as well as other elements present. As such, the resulting iron can be classified as steel having a carbon content of between 0.01-2%, cast iron exhibiting a carbon content of more than 2 but less than 6.67%, or stainless steel with chromium content more than 10%. Noteworthy, for steels, the ductility and toughness decrease with an increase in carbon concentration. Other significant “metallic alloys are those of aluminum, titanium, copper and magnesium” (Day 1988). The scope of this paper, however, centers on steel, aluminum, and copper. As such, this paper highlights the metals separately with respect to their physical properties and application.
Also known as carbon steel, steel is an alloy with the main trace elements being carbon. The degree of carbon should however not be more than 2%. In essence, “the term carbon steel may be used to refer to steel that is not stainless steel” (Bray 1947). The degree of carbon concentration alters the physical properties of steel. For instance, with an increased carbon concentration steel becomes less ductile, hard and stronger, and reduces welding ability. Nevertheless, the melting point decreases. Basing on the “carbon concentration, steel can be classified into four categories: low and mild carbon steel, medium carbon steel, high carbon steel and ultra-high carbon steel” (Bauccio 2005)
Low and mild carbon steel represent steel with the lowest carbon concentrations and the most commonly used in the market. Basically, for these types, the carbon concentration is shy of 0.6%. Vitally, low carbon steel (or A36) has a carbon concentration of approximately 0.05-0.3% while the mild carbon steel (1040) exhibits a concentration of roughly 0.3-0.6%. As a consequence, this enhances the metal’s malleability and ductility characteristics. However, of the two, mild steel exhibits relatively low tensile strength. Nevertheless, low carbon steel “suffers from yield-point run-out where the material has two yield points” (Bray 1947). In essence, this is “accounted for by the fact that the upper yield point is relatively higher than the second thus the yield drops dramatically after the first” (Walker 1998). Vitally, because of the low carbon concentration, they are easy to cold-form and hence easy to handle. Of note, for all the low carbon steels the modulus of elasticity and the poison’s ratio is the same (207 GPa and 0.3 respectively). Nonetheless, the tensile strength varies depending on the annealing temperatures and whether the metal is hot or cold rolled. To this end, A36 has a tensile strength of approximately 400-500 MPa while 1040 has roughly 520-590 MPa (Harry 1997).
With a carbon content range of approximately 0.3-1.7%, carbon steels can successfully undergo heat-treatment. This group under the umbrella of high carbon steels encompasses the medium, high, and ultra-high carbon steels. Significantly, the trace elements added functions to modify the properties of the carbon steel. For instance, “sulfur makes steel crumbly and brittle at working temperatures” (Walker 1998). Moreover, manganese often increases the ‘hardenability’ of these steels. Narrowing down to the specifics, medium carbon steel boasts a carbon content of approximately 0.3-0.59% by weight. As such, it balances strength and ductility, and it exhibits good wear resistance boosting its applicability in automotive. With a carbon content of approximately 0.6-0.99% (4140), high carbon steel exhibits relatively high strength thus, it finds application in springs (tensile strength is roughly 655-1720 respectively). Finally, ultra-high carbon steel (1-2% carbon content) exhibits unprecedented hardness, and hence, it finds application, for example, in axels and knives (Ashby & David 1992).
Among the world’s most common elements aluminum comes third to oxygen and silicon respectively. Noteworthy, embedded within granite of the earth’s crust, aluminum accounts for 8.1% by weight of the total earth’s crust. Principally, it is present in massive quantities in both feldspar and mica of the granite. Pure aluminum is a distinct metal that is uniquely applied in diverse fields. In essence, whatever makes aluminum distinct are its physical properties. Basically, “It is a soft, light, and gray metal that resists corrosion when pure in spite of its chemical activity because of a thin surface layer of oxide” (Egarmo, Black & Kohser 2003). Moreover, it typifies both malleability and ductility characteristics that make it unsuitable for structural use. Ideally, Young’s modulus “of pure aluminum is 10 x 106 psi, the shear modulus 3.8 x 106 psi, Poisson’s ratio 0.33, and the ultimate tensile strength 10,000 psi, with 60% elongation” (Harry 1997). With these properties, it finds application, for example, piping, cable sheathing, and electric conductors.
Aluminum is alloyed with copper to modify its physical characteristics. For instance, when alloyed with 3.5-5% copper (Al 2014 or HE15), it exhibits a tensile strength of approximately 30,000-63,000 psi. Moreover, it is relatively corrosive free, has the best ‘machinability’ enhanced by annealing temperatures of 750-800 F for a span of 2 hours, and is fairly workable. With these properties, it finds application, for example, in screw machines, truck tire rims, and rivets (Groover 2007).
When aluminum is alloyed with approximately 0.4-0.9% Mg, 0.3-07% Si, 0.6% Fe, 0.3% Mn, and 0.1% Cu, then the alloy (HE9) exhibits suitable properties vital in, for example, architectural foundation and furniture. Basically, depending on the annealing temperatures, the tensile strength does not exceed roughly 107 psi (Smith & Hashemi 2006).
Cartridge Brass C26000 (70/30 Brass 9) is a name given to copper alloy by virtue of its application in making ammunition casing. It is basically composed of brass and copper mixed at a ratio of 30 to 70. Cartridge brass finds application in the diverse mechanical field thanks to its unique physical properties. To this end, the alloy is popular for it displays abilities to resist corrosion and wear, has a good damping characteristic, has moderate strength, and it retains spring. Moreover, it is the most ductile of all brasses. Noteworthy, it has a tensile strength of 16 MPa psi, poison ratio of 0.35, and thermal conductivity of 120 W/m/K (Adelbert 1992).
Experimental Procedure
In this experiment, a tensile test machine (Zwick-Roell 1474) was used. The materials to be tested were steels (at 0.1, 0.2, 0.4, 0.8, 1.15%), pure aluminum and its alloys (Al HE9 and Al HE15), and 70/30 brass (cartridge brass). With the aid of software, the parameters recorded were stress, percentage strain, force applied, and elongation. From this, a graph of stress against percentage strain was automatically plotted from which the maximum stress, force, elongation at a maximum force, and elongation just before breaking were determined.
Results
Table 1: of the parameters measured on steel samples.
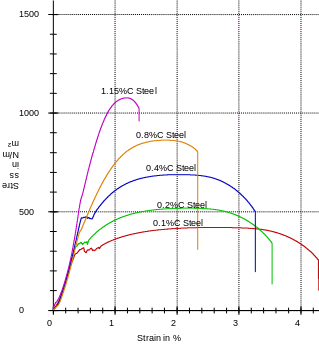
Table 2: of the parameters measured on aluminum and brass samples.
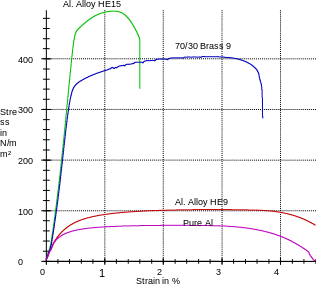
Discussion
The essence of performing this experiment was to partly analyze metal and metal alloys and hence correlate them with their application and, on the other part, to relate them and ascertain whether they concur with the literature values. In a view of the aforementioned objective a graph of stress against percentage strain was plotted, vital in determining the relative physical characteristics of the samples.
Graph 1 represents a plot of values obtained after analyzing carbon steels. As portrayed by the graph, it is evident that the tensile strength of carbon steel increase with an increase in carbon concentration. As such, more strength is applied to 1.15% than 0.1% carbon steel to break. From this analysis, we can appreciate the application of 1.15% carbon steel (ultra-high carbon steel) in the making of, for example, axel and knives which require strong materials. However, it has a low ‘weldability.’ Still on the same graph, another physical property is manifested- ductility. From the same graph, one can easily tell that ductility and malleability in carbon steel decrease with an increase in carbon content (Jones 1949). To this end, we can appreciate the application of low carbon steel in sheeting as well as in structure formation thanks to its amenability to tooling and welding. On comparing the experimental and the literature values, one word dominates consistency. For instance, when focusing on the tensile strength and on converting the tabulated data to the SI units (N/m2), the results tally with the literature values.
As for graph 2, apart from aluminum and its alloys, copper alloy (70/30 Brass) is incorporated. From this perspective, the physical properties of the samples can easily be analyzed and compared. From the trends typified by the graph, it is apparent that Al. Alloy HE15 is the strongest of the whole samples. On the other hand, pure aluminum is the weakest of all and among the most ductile. To this end, with its ductility and malleability, pure aluminum is unsuitable for structure formation. Similarly, we can appreciate the use of Al. Alloy HE15 information of, for example, screw machines, truck tire rims, and rivets. When focusing on the trend of 70/30 brass, it is clear that the sample is relatively strong and ductile. As such, we can appreciate its application in spring as well as cartridge casing manufacture. Just like pure aluminum, Al. Alloy HE9 is relatively weak and ductile. Therefore, it is best suited for making furniture.
The experimental data are consistent with the tabulated literature values for all the samples. Therefore, the experiment was near-perfect.
Conclusion
The objective of this experiment was to partly analyze metal and metal alloys and hence correlate them with their application and, on the other part, to relate them and ascertain whether they concur with the literature values. From the data and the trends obtained, the objectives of the experiment were realized since trends marched with the specific application, and the values obtained were consistent with the literature values.
References
Adelbert, PM 1992, Materials of Construction: Their Manufacture and Properties, University of Wisconsin Press, Madison.
Alexander, W & Street, A 1976, Metals in the Service of Man, 6th ed., Pelican Books, London.
Ashby, M F & David, J 1992, “Chapter 12”. Engineering Materials 2 (with corrections ed.), Pergamon Press, Oxford.
Bray, JL 1947, Non-Ferrous Production Metallurgy, John Wiley & Sons, New York.
Bauccio M 2005, ASM metals reference book, ASM International 2005, New York.
Day, J 1988, “The Bristol Brass Industry: Furnaces and their associated remains,” Journal of Historical Metallurgy, vol. 22, no. 1, pp. 24.
Egarmo, E, Black, J T & Kohser, A 2003, Materials and Processes in Manufacturing (9th ed.), John Wiley & Sons, New York.
Groover, M P 2007, Fundamentals of Modern Manufacturing: Materials, Processes and Systems (3rd ed), John Wiley & Sons Inc., Hoboken, NJ.
Harry, A M 1997, The economy of later Renaissance Europe, 1460–1600, Cambridge University Press, Cambridge.
Hashemi, J 2001, Foundations of Material Science and Engineering (4th ed.), McGraw-Hill, London.
Higgins, RA 1971, Engineering Metallurgy, 3rd ed., The English Universities Press, London.
Jones, WN 1949, Inorganic Chemistry, Blakiston Press, Philadelphia.
Simons, EN 1970, A Dictionary of Alloys, Cornell University Press, Kentucky.
Smith, F & Hashemi, J 2006, Foundations of Materials Science and Engineering, Routledge, London.
Walker, R 1998, “Mass, Weight, Density or Specific Gravity of Different Metals”. Density of Materials, Pergamon Press, Oxford.