Most chemical reactions are reversible. During a chemical reaction, reactants are usually converted into products. However, some products turn back into reactants at the same time. Chemical equilibrium can be described as a state in a reversible reaction where the speed of the forward reaction is equal to the rate of the reverse reaction (Liu, Liu and Drew 2015). At this stage, there are equal concentrations of products and reactants. The quotient of concentration of product over the concentration of reactants is constant. An equilibrium constant is a parameter that is calculated using the concentrations of each side of an equation when the reaction reaches equilibrium. Several factors can affect the chemical equilibrium of a system, including alterations in volume, pressure, temperature and the presence or absence of a catalyst. Le Chatelier’s principle, also known as “The Equilibrium Law” is useful in forecasting the impact of altering specific conditions on chemical equilibria (Torabi and Abbasi 2017). It states that for a system in equilibrium, the application of any constraints results in a change in equilibrium to offset the effects of the constraint. All reversible reactions follow Le Chatelier’s principle, which means that when a certain condition changes, the reaction will not be at equilibrium for a short while. Thereafter, it will return to equilibrium after countering the factor that caused the initial shift in equilibrium.
Impact of Equilibrium Systems/Process on Biological, Biochemical and Technological Processes
Factors that affect equilibrium are manipulated technologically in the manufacture of various industrial chemicals, including the production of lime from limestone, the manufacture of methanol, ethanol and production of sulphuric acid (Tejeda-Iglesias et al. 2016). In biological and biochemical systems, equilibrium reactions are applied in metabolic reactions where enzymes catalyse the production of different products from substrates. For example, glucose is maintained at constant concentrations despite being used in large quantities to provide the energy needed to sustain life. Therefore, the body replaces used up glucose by digesting ingested carbohydrates. Similarly, when glucose levels are very high, such as after a meal, the body maintains normal levels of glucose by storing excess glucose as glycogen.
Production of Sulphuric Acid and Equilibrium
Sulphuric acid, one of the most known inorganic acids in the world, has numerous benefits. It is an oily, colourless liquid with no odour. Sulphuric acid is an essential component of batteries, water treatment plants and ore production. It is also widely used in the production of fertilizers. Approximately 65% of fertilizers in the world is made of this acid combined with other chemicals.
The production of sulphuric acid involves a reversible reaction that follows Le Chatelier’s principle (Apodaca, d’Aquin and Fell 2017). Manufacturers use the rule to change certain conditions to improve the yield of the product. This paper discusses the equilibrium process in the industrial manufacture of sulphuric acid.
The contemporary way of producing sulphuric acid in the industries is the contact process, which has replaced older methods such as the lead chamber process. In this approach, sulphuric acid is produced through three key steps the first of which is the oxidation of sulphur to form sulphur dioxide. The ensuing product is then converted to sulphur trioxide in a reversible reaction. The third step entails adding water to sulphur trioxide to form sulphuric acid.
Sulphur dioxide is made by the combustion of sulphur in excess air. The chemical equation for the reaction is as follows:
S (s) + O2 (g) → SO2 (g).
An alternative way is by heating sulphide ores such as pyrite (FeS2) in excess air as shown in the following reaction:
FeS2 (s) + O2 (g) → Fe2O3 (s) + SO2 (g).
Excess air is used to ensure the complete combustion of sulphur into its oxide.
The formation of sulphur trioxide is a reversible, exothermic reaction. Exothermic reactions are accompanied by the generation of heat. In this case, 196 kJ of heat is produced for every mole of sulphur trioxide produced.
SO2 (g) + O2 (g) ⇌ SO3 (g). ΔH= -196 kJ mol-1
This process occurs in the presence of solid vanadium (V) oxide as a catalyst. Vanadium is a transition metal that changes its oxidation states in the course of the reaction but remains unaltered by the end of the entire process. Vanadium (V) oxide oxidises sulphur dioxide to sulphur trioxide and becomes vanadium (IV) oxide (Erust et al. 2016).
SO2 + V2O5 (s) → SO3 (g) + V2O4 (s)
However, in the presence of oxygen, vanadium (IV) oxide is re-oxidised back to vanadium (V) oxide.
V2O4 (s) +1/2 O2 (g) → V2O5 (s)
Other conditions necessary for this reversible reaction are a pressure of 1 to 2 atmospheres and a temperature ranging from 400 to 450oC. Furthermore, the ratio of oxygen to sulphur dioxide should be maintained at 1:1. The process for this reversible reaction is illustrated in figure 1 below.
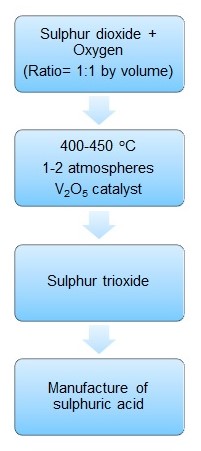
The last step involves converting sulphur trioxide into sulphuric acid by the addition of water. However, this step is not as simple and straightforward as it appears. Adding water directly to sulphur trioxide creates a fog. To avoid this occurrence, sulphur trioxide is first liquified in concentrated sulphuric acid to form a compound known as oleum or fuming sulphuric acid (Tejeda-Iglesias et al. 2016).
H2SO4 (l) + SO3 (g) → H2S2O7 (l)
Oleum is then dissolved with water to form twice as much concentrated sulphuric acid as shown in the following reaction.
H2S2O7 (l) + H2O (l) → 2H2SO4 (l)
Analysis of Conditions Necessary for Optimal Functioning of the Equilibrium
The Ratio of Sulphur Dioxide and Oxygen
As stated before, the volume ratio of these constituents should be equal.
Avogadro’s Law implies that at the same conditions of temperature and pressure, equal volumes of gases have the same number of molecules. However, going by the chemical reaction, this translates into excess oxygen. Based on Le Chatelier’s principle, excess oxygen will shift the equilibrium to the right, thereby leading to the formation of more products (Liu, Liu and Drew 2015). Consequently, the yield can be increased cost-effectively because air is a cheap raw material.
Temperature
Producing the maximum yield of sulphur trioxide requires the equilibrium to be shifted to the right as far as possible. The negative sign of the enthalpy of the reaction shows that the forward reaction is exothermic. Based on Le Chatelier’s Principle, equilibria involving exothermic reactions are usually favoured by reducing the temperature (Torabi and Abbasi 2017). As a result, the system reacts to the reduction in temperature by producing more heat, which is achieved by the forward reaction. By industrial standards, 400 to 450°C is the lowest temperature that pushes this reaction to the right in the shortest time. Even though the above temperatures appear high, a further reduction in temperature slows down the reaction significantly and is not feasible.
Pressure
The equilibrium reaction has 3 molecules on the left and 2 molecules on the right. Increasing the pressure of a system is known to shift the equilibrium towards a reaction that favours the production of fewer molecules (Torabi and Abbasi 2017). In this case, an increase in pressure would lead to the production of more sulphur trioxide. However, the few molecules would, in turn, cause a decline in pressure. Therefore, to increase the reaction rate and maximise the yield, high pressures need to be applied to the system. It was noted that the pressures used are close to atmospheric pressure. The rationale for this feature is that the production of high pressures is an expensive process. Since 99.5% conversion of reactants to products is possible at near atmospheric pressure, it makes economic sense to maintain these low pressures.
Catalyst
Catalysts do not influence the position of the equilibrium. However, they increase the rate at which equilibrium is attained. Without vanadium (V) oxide, no noticeable reaction happens (Apodaca, d’Aquin and Fell 2017). However, the inclusion of the catalyst establishes dynamic equilibrium in a short time. Other examples of industrial manufacturing processes that take advantage of the above-mentioned principles of equilibrium are the synthesis of ammonia via the Haber process (Liu, Liu and Drew 2015), methanol production (Huang et al. 2015), and lime formation.
In conclusion, the industrial manufacture of sulphuric acid through the contact process involves three main steps. The second step is reversible and is governed by the principles of Le Chatelier’s principle. Maintaining a pressure of 1 to 2 atmospheres, a temperature of 400 to 450oC and equal volumes of reactants in the presence of vanadium (V) oxide catalyst leads to the production of appreciable volumes of products in a reasonable time.
References
Apodaca, Lori E., Gerard E. d’Aquin, and Robert C. Fell. 2017. “Sulfur and Sulfuric Acid.” In Handbook of Industrial Chemistry and Biotechnology, 1241-66. New York: Springer.
Erust, Ceren, Ata Akcil, Zyuldyz Bedelova, Kuanysh Anarbekov, Aliya Baikonurova, and Aysenur Tuncuk. 2016. “Recovery of Vanadium from Spent Catalysts of Sulfuric Acid Plant by Using Inorganic and Organic Acids: Laboratory and Semi-Pilot Tests.” Waste Management 49: 455-61.
Huang, Men-Han, How-Ming Lee, Kuo-Chao Liang, Chin-Ching Tzeng, and Wei-Hsin Chen. 2015. “An experimental study on single-step dimethyl ether (DME) synthesis from hydrogen and carbon monoxide under various catalysts.” International Journal of Hydrogen Energy 40(39): 13583-93.
Liu, Ying, Yue Liu, and Michael GB Drew. 2015. “A Mathematical Approach to Chemical Equilibrium Theory for Gaseous Systems IV: A Mathematical Clarification of Le Chatelier’s Principle.” Journal of Mathematical Chemistry 53(8): 1835-70.
Tejeda-Iglesias, Manuel, Jason Szuba, Ron Koniuch, and Luis Ricardez-Sandoval. 2018. “Optimization and Modeling of an Industrial-Scale Sulfuric Acid Plant under Uncertainty.” Industrial & Engineering Chemistry Research 57(24): 8253-266.
Torabi Rad, M., and Afshin Abbasi. 2017. “The Conditions of the Violations of Le Chatelier’s Principle in Gas Reactions at Constant T and P.” Iranian Journal of Mathematical Chemistry 8(1): 47-52.