Introduction
Gas chromatography (GC) is a technique used to separate volatile components of a complex mixture. The method employs a column made of a flow-through narrow tube in which a sample containing various chemical components passes in a gas stream. The gas stream in chromatography is referred to as a mobile phase. The different components in the gas stream interact with a certain column filling referred to as a stationary phase depending on their chemical and physical characteristics.
The individual chemicals are then detected and identified electronically as they exit the column. Different parameters are employed to ensure the successful separation of exiting gases at different retention times. This includes the nature of the stationary phase, carrier gas flow rate, temperature, and column length. The use of gas chromatography in forensic applications has gained popularity mainly due to the versatility, reliability, and sensitivity of the technique. In crime laboratories, gas chromatography, together with other models like mass spectrometry and infrared spectroscopy, has helped solve unique crime-related problems (Amirav, Gordin, Poliak, Alon & Fialkov, 2008).
It is easy to use and has simple instrumentation and automation that allows for easy interpretation of data. This means that the technique will continue to be used for some time. The focus of this paper will be on the role of gas chromatography within forensic applications. The essay will elaborate on the analytical approaches used the challenges faced and some of the current solutions.
Forensic science encompasses many fields of science and creates a connection between their applications and law. In most cases, it entails using chemistry and related science principles to the examination of physical evidence obtained from analyzing samples collected from crime scenes (Houck & Siegel, 2010). The information is then interpreted by forensic experts and can be used as part of the evidence in a court of law. However, gas chromatography is not applicable in all forensic analyses. The most common areas of application include toxicological analyses in blood and drug abuse. The technique is also widely applied in criminalistics that includes analysis of explosives, trace evidence of fibers, paints and other polymers and debris from fire scenes (Bogusz, 2008).
Analytical Approaches Used
In almost all forensic applications of gas chromatography, the sample under study is prepared by dissolving it in a suitable solvent. The formed solution is then introduced into the chromatograph instrument by use of a syringe. The analysis of a suspected compound is carried out by the use of a comparative substance known as a standard. For instance, to analyze a sample suspected to be a drug such as hashish, a small ration is dissolved in an appropriate solvent such as methanol, chloroform, or methylene chloride (Barry & Grob, 2004). The sample is then introduced into the heated injector port of the chromatogram instrument.
The carrier gas, which in most cases is helium, nitrogen, or hydrogen constituting the mobile phase, is also introduced into the injector port. The liquid sample introduced is volatilized due to the high temperature in the port. The carrier gas carries with it the volatilized sample. In addition, this gas introduces its sample into the stationary phase where chromatographic separation occurs. The sample under analysis, hashish, emerges from the column in a certain retention time. This is then compared to the known standard of the sample under analysis. One can positively identify the compound under study if it is part of the introduced sample by studying the retention time and the information recorded on the electronic detector (Scheig, 1970).
When the compound of analysis is solid and cannot easily dissolve in conventional solvents, an alternative approach known as pyrolysis is employed. Here, the solid sample is introduced to high temperatures until it decomposes into a gaseous substance. This is most commonly used for products such as polymeric fibers and paint chips collected from crime scenes. The gaseous state of the compound can now then be introduced into a gaseous chromatography column. Gas chromatography employs the use of various detectors. However, the most commonly used detector in the field of forensic science is mass spectrometry, nitrogen-phosphorus, and flame ionization (Bell, Fisher & Shaler, 2008).
The mass spectrometry detector can give a definitive identity of compounds under study, as well as accurately determine the sample quantity. This meant that the detector was most preferred in the majority of forensic applications. A flame ionization detector is mostly applicable in determining samples related to arson cases mainly due to its increased sensitivity to hydrocarbons. In almost all incidents of arson cases, the accelerants have hydrocarbons as the primary constituent (Muller, Levy & Shelef, 2011).
On the other hand, Nitrogen-Phosphorus Detectors are mostly used in toxicological tests and drug analysis. The gas chromatography technique is preferred over other methods in forensic applications. This is because it can accurately provide information on the actual identity of the compound if the appropriate standard is used. This qualitative information is vital particularly in cases of complex criminal investigations. The capability of the technique to provide quantitative data concerning a sample is vital. This is especially in toxicological studies where such information can be used by physicians while making necessary recommendations on an intoxicated patient.
This is also important in drug analysis studies where gas chromatography helps accurately determine the quantities of the different components making up a sample. In such cases, the technique is used to detect whether the compound under analysis is pure for instance in drug syndicate incidents, and this can provide vital evidence for use in a criminal case. Gas chromatography can facilitate the formulation of a chemical identity or signature of the compound under study by utilizing a minute sample collected as evidence in a criminal case (Savchuk, Barsegyan, Barsegyan & Kolesov, 2008).
Interpreting the data from the gas chromatography analysis is one of the most important steps. This leads to the gas chromatography instrument being connected to the detector. The connection between gas chromatography and a mass spectrometer is the most common of these combinations. The mass spectrometer facilitates the performance of a full scan or a selected ion monitoring with modern GC-MS instruments performing such functions concurrently or separately. The spectrum generated offers a guide on the concentration of the sample under analysis. The spectrum gives analytical information in both original and comparative formats (de Vos et al, 2011).
The information can be relayed in two ways. In one way, the spectrum generated is compared with a library of earlier studied spectra that serve as standards. Computers are now a days used to give accurate information by displaying the data on the spectrum scales that are easily readable in digital displays. Computer programs have been developed that can simultaneously correlate data such as between retention times. The other method involves measuring the peaks of another.
The peaks are assigned different values and are associated with different chemical or physical characteristics of the different compounds. Selective ion monitoring entails monitoring only the peaks that are closely associated with the suspected analyte. This is based on the knowledge that related ions such as isotopes will have almost similar or closely related retention times (Mühlen & Marriott, 2012). The methods have been in use within forensic applications, and the results obtained were found reliable and efficient.
An example of the application of gas chromatography is in the analysis of body fluids. This is attributed to the ability of the technique to give accurate results when analyzing non-polar compounds. In analyzing blood samples such as serum or plasma, the technique can easily detect psycho-pharmaceuticals found in the blood in detectable amounts (Varlet, De, Croutte, Augsburger & Mangin, 2012).
For urine to be analyzed through gas chromatography, it requires to undergo derivatization since it contains a myriad of polar metabolites. In such analysis, a comprehensive screening protocol is required to ensure the sample is well prepared. For products such as the urine sample, derivatization improves the quality of detection especially for compounds containing hydroxyl and amino end groups such as phenols, amines, and imines (Halket & Zaikin, 2006). Use of solvent extraction methods that utilize compounds such as butyl acetate and butyl chloride help in fractionating the basic, neutral and acidic drug compounds. These can then be readily introduced into the gas chromatography equipment for analysis. With such a preparation procedure, the technique can record high levels of accuracy in detection (Casale, Colley & LeGatt, 2012).
The application of gas chromatography in criminal forensics has gained a lot of interest in America. There is the American Society for Testing Materials (ASTM), which carries out analysis on fire debris in arson suspect scenes. ASTM utilizes the gas chromatography technique for detection and identification. Detection of illegal narcotics and poison detection in toxicological analysis in biological specimens today largely employs gas chromatography owing to its high level of accuracy. Security systems in many nations are employing GC-MS technology in detecting explosives especially in airports (Cook, LaPuma, Hook & Eckenrode, 2010). Medical detectives employ GC-MS techniques to analyze metabolic and congenital disorders in a myriad screening test as well as in crime scene analyses (Watterson & Desrosiers, 2011).
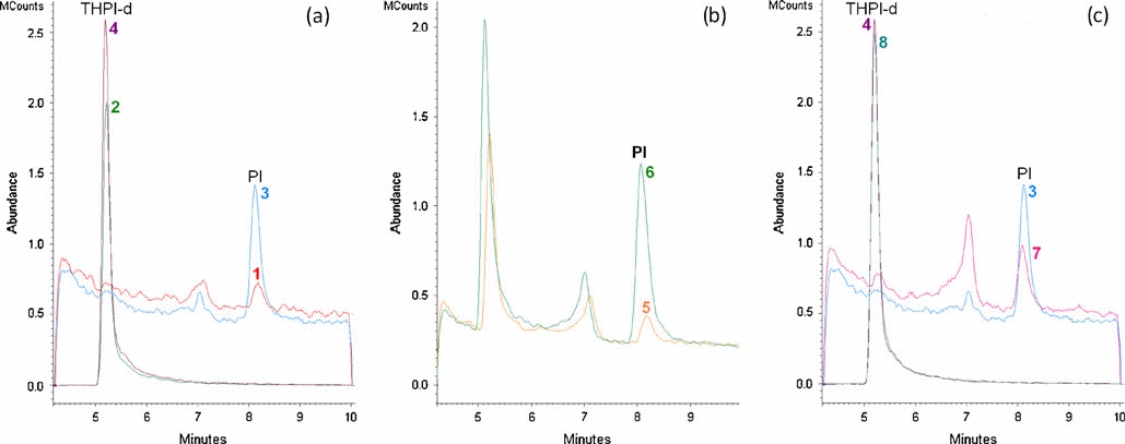
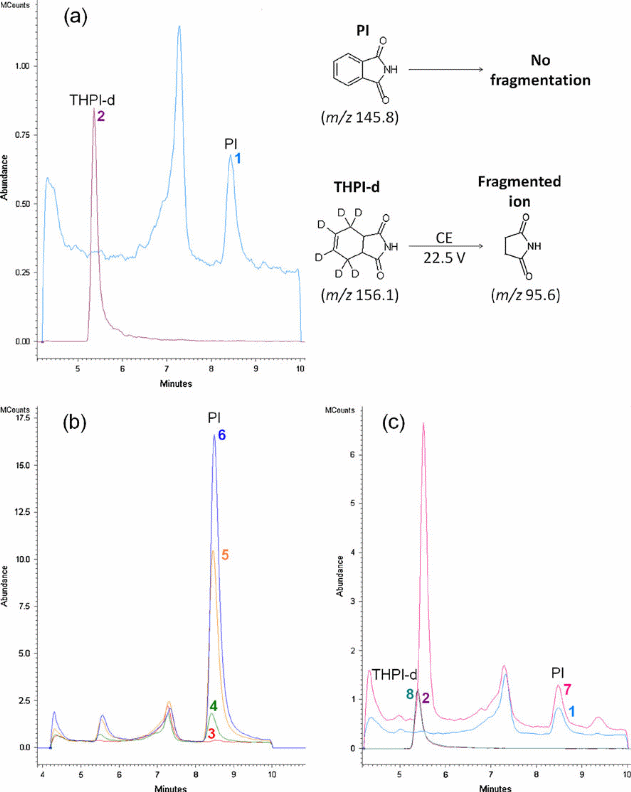
Challenges Faced when using Gas Chromatography
The major shortcomings associated with gas chromatography include a poor performance by the GC screen. Since the instrument requires heat to allow volatilization of analytical compounds, the method may not be applicable in the analysis of some thermally labile components. The temperature of the heat must also be within acceptable ranges as it can affect the performance of column packing. In other cases, some analytes are adsorbed irreversibly into the chromatographic system.
This makes analysis a complex and time-consuming process. The system can analyze complex mixtures, and this leads to a shortcoming in the ability to ensure the system is functioning optimally and generating accurate results (Stauffer, Dolan & Newman, 2008). When the gas chromatography uses a Nitrogen Phosphorus detector, it cannot give accurate results when putrid analytes are introduced. These products in most cases contain organic bases which when in high concentrations are known to affect the performance of a Nitrogen-Phosphorus detector rendering the technique ineffective. The gas chromatography machine is expensive. Furthermore, its operation requires expertise training increasing the cost of its use. The system is also slower in sample analysis as compared to other chromatography techniques (Burleson, Gonzalez, Simons & Yu, 2009).
Current Solutions
The challenges encountered when working with the gas chromatography technique in forensic applications emanate from sample preparation methodology, the physical and functional parameters of the GC equipment, and the interpretation of results. Highly selective methods, targeting one or few analytes, are in application today to overcome the problems in the error-prone sample preparation stage (Moroni et al, 2010).
New solvents, membranes, and columns have been designed to increase efficiency. The use of the online preparation stage allows automation and system integration to ensure the preparation of the analyte and result generation is matched (Koning, Janssen & Brinkman, 2009). The GC instrument parts have been undergoing modifications in line with modern-day inventions to increase efficiency. One part targeted is the column with the length and breadth undergoing changes to decrease the time for analysis. Shorter columns have been found to reduce analysis time to increase efficiency. Adjustments in column temperature based on theoretical research data have informed the development of efficient GC equipment.
The gas chromatography equipment in use today has undergone many changes including automation. The computer-controlled equipment generates real-time accurate data displayed on computer screens. This is a major shift from earlier models that depended on the visual acuity of the expert to interpret data. Of course, this method was prone to deviations due to human and parallax errors (Masˇtovska´ & Lehotay, 2005).
Conclusion
The use of gas chromatography in forensic applications has greatly transformed the field of forensic sciences. The accuracy and efficiency of the technique have informed its widespread use in applications such as toxicological tests, analysis of explosives, trace evidence of fibers, paints and other polymers, and debris from fire scenes. However, the technique faces some technical challenges that may affect the accuracy of results. Nevertheless, advancements in research and technology have enabled continued adjustments to increase the efficiency and accuracy of the technique, and as evidence shows, the role of GC within forensic applications remains relevant.
Reference List
Amirav, A, Gordin, A, Poliak, M, Alon, T and Fialkov, AB 2008, Gas Chromatography Mass Spectrometry with Supersonic Molecular Beams, Journal of Mass Spectrometry 43: 141–163.
Barry, EF & Grob, RL, 2004, Modern practice of gas chromatography, Wiley-Interscience, Hoboken, NJ.
Bell, S, Fisher, BAJ & Shaler, RC 2008, Encyclopedia of forensic science, Facts On File, New York, NY.
Berthet, A, Bouchard, M, Schüpfer, P, Vernez, D, Danuser, B & Huynh, C 2011, Gas chromatography-tandem mass spectrometry (GC/APCI-MS/MS) methods for the quantification of captan and folpet phthalimide metabolites in human plasma and urine. Analytical & Bioanalytical Chemistry, 399(6), 2243-2255.
Bogusz, MJ 2008, Forensic science, Elsevier, Amsterdam.
Burleson, G, Gonzalez, B, Simons, K & Yu, J 2009, Forensic analysis of a single particle of partially burnt gunpowder by solid phase micro-extraction–gas chromatography-nitrogen phosphorus detector, Journal of Chromatography A, 1216, 22, pp. 4679-4683.
Casale, J, Colley, V, & LeGatt, D 2012, Determination of Phenyltetrahydroimidazothiazole Enantiomers (Levamisole/Dexamisole) in Illicit Cocaine Seizures and in the Urine of Cocaine Abusers via Chiral Capillary Gas Chromatography-Flame-Ionization Detection: Clinical and Forensic Perspectives, Journal Of Analytical Toxicology, 36, 2, pp. 130-135.
Cook, G, LaPuma, P, Hook, G & Eckenrode, B 2010, Using Gas Chromatography with Ion Mobility Spectrometry to Resolve Explosive Compounds in the Presence of Interferents, Journal of Forensic Sciences, 55, 6, pp. 1582-1591.
de Vos J, et al 2011, Comprehensive two-dimensional gas chromatography time of flight mass spectrometry (GC×GC-TOFMS) for environmental forensic investigations in developing countries, Chemosphere, 82, 9, pp. 1230-1239.
Halket JM & Zaikin VG 2006, Derivatization in mass spectrometry –7. On-line derivatisation/degradation, European Journal of Mass Spectrometry 12, 1, pp. 1–13.
Koning S, Janssen H & Brinkman U 2009, Modern Methods of Sample Preparation for GC Analysis. Chromatographia, 69(1): 33-78.
Masˇtovska´, K & Lehotay S 2005, Practical approaches to fast gas chromatography–mass spectrometry. Journal of Chromatography, 1000 (1-2): 153-180.
Houck, MM & Siegel, JA 2010, Fundamentals of forensic science, Academic Press, Burlington, MA.
Mühlen, C & Marriott, P 2012, Retention indices in comprehensive two-dimensional gas chromatography, Analytical & Bioanalytical Chemistry, 401, 8, pp. 2351-2360.
Moroni, R, et al 2010, Statistical modelling of measurement errors in gas chromatographic analyses of blood alcohol content, Forensic Science International, 202, 1-3, pp. 71-74.
Muller, D, Levy, A & Shelef, R 2011, Detection of gasoline on arson suspects’ hands, Forensic Science International, 206, 1-3, pp. 150-154.
Savchuk, S, Barsegyan, S, Barsegyan, I & Kolesov, G 2008, ‘Chromatographic study of expert and biological samples containing desomorphine’, Journal Of Analytical Chemistry, 63, 4, pp. 361-370.
Scheig, RL 1970, Gas Chromatography in Biology and Medicine, The Yale journal of biology and medicine, 43(2): 122-123.
Varlet, V, De Croutte, EL, Augsburger, M & Mangin, P 2012, Accuracy profile validation of a new method for carbon monoxide measurement in the human blood using headspace-gas chromatography–mass spectrometry (HS-GC–MS), Journal Of Chromatography B: Analytical Technologies In The Biomedical & Life Sciences, 880, pp. 125-131.
Stauffer, E, Dolan, JA & Newman, R 2008, Fire debris analysis, Academic Press, Boston, Mass.
Watterson, J & Desrosiers, N 2011, ‘Examination of the effect of dose-death interval on detection of meperidine exposure in decomposed skeletal tissues using microwave-assisted extraction’, Forensic Science International, 207, 1-3, pp. 40-45.