How solar panels operate
A solar panel, also known as a photovoltaic panel, is an assemblage of photovoltaic cells that convert sun rays directly into electricity through a process known as the photovoltaic effect; the interconnected photovoltaic cells (or the photovoltaic module) are protected from the environment, sometimes with a glass covering and backed with fiber-glass, metal, or plastic. Therefore, their operation is based on the photovoltaic effect, where the photovoltaic cells make use of the light energy’s photons to generate electricity.
To generate electricity, the photovoltaic cells (also known as solar cells) absorb photons from light energy. It is worth noting that sunlight is made up of packets of solar energy known as photons and that these photons hold varying energy amounts depending on the various wavelengths of the sun rays spectrum. Therefore, when photons hit a photovoltaic cell, they are either absorbed, reflected, pass through the cell. The photons that are absorbed generate electric current.
When the photovoltaic cells absorb the light energy, the energy contained in the photons is transmitted to an electron of an atom of the cell; the photovoltaic cell is a semiconductor. With this energy, the electron breaks away from its usual location associated with the semiconductor atom to become an electric current within an electrical circuit. Through special properties of the photovoltaic cells, the electric field built within provides the driving force (or voltage) for the electric current to pass through external loads (Sandia National Laboratories).
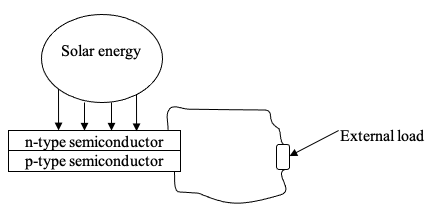
Solar panels in the market
There are three basic types of photovoltaic (solar) panels in the market, distinguished by the kind of solar cells constituting them. These include the amorphous or thin-film cell photovoltaic panels, the crystalline silicon photovoltaic panels, and the polycrystalline cells panels.
The thin-film solar cell technology is widely used to manufacture most commercial solar panels for various applications (Hirshman, Hering, Schmela 2008). These cells are made with a thin silicon film deposited onto a folio of different materials like steel. The photovoltaic panel is created as a single piece without the individual photovoltaic cells being visible. These types of solar panels are not very efficient, although they are more cost-effective in regards to their cost per watt rating. Nonetheless, their power density is also low, meaning that more solar panels are required to give the same power as maybe solar panels based on crystalline solar cells. In effect, their commercialization has adamantly remained difficult (Hirshman, Hering, Schmela 2008). As of 2007, the production of cadmium-based solar panels was 4.7% of the total market share, while that of thin-film silicon was 5.2% and that of copper indium gallium selenide was 0.5% (Hirshman, Hering, Schmela 2008). The common energy conversion efficiency of most panels in this category is about 10% (Creative Commons 2002), which is very low in relation to the other two types. With developments of this technology, however, manufacturers have managed to produce thin-film-based solar cells with an efficiency of about 19.3% (Ramanathan, Teeter, Keane, Noufi 2005; Solar Energy Alliance), with the solar module having an efficiency of about 17.2% (Sanyo Energy Corp. 2009).
Crystalline silicon solar panels are made up of single-crystalline silicon solar cells that are connected in series. Averagely, one cell gives out a voltage of about 0.5 volts to 0.6 volts. These types of solar modules have a longer lifespan when compared to that of thin-film solar modules. They have a maximum energy conversion efficiency of about 25% “because they are most sensitive to infrared radiation,” whose energy is relatively low (Creative Commons 2002). Moreover, in theoretical terms, the crystalline silicon modules have somewhat higher efficiency than the polycrystalline, although practically, their performance is more or less the same.
The polycrystalline solar panels are composed of solar cells made out of several crystal silicon. Their “efficiency is limited to about 20% due to internal resistance at the boundaries of the silicon crystals” (Creative Commons 2002).
Initial calculations to operate 85 and 100-kilo watts
Generally, the performance of solar panels or solar modules is measured under test conditions, of which the standard test conditions normally used is a temperature of 250 C, the irradiance of 1000 W / m2 (watts per square meter), the solar spectrum of (air-mass) AM 1.5. The electrical properties rated include the open-circuit voltage, nominal power, short circuit current, maximum power current, maximum power output, and efficiency of the module in percentage.
The percentage of the amount of power transformed from light photons energy into electrical energy by photovoltaic cells is denoted by its conversion efficiency. It involves the maximum power output (Pm), irradiance (E), and the solar panels surface area (Ac) under the standard test conditions. Thus, the conversion efficiency of the photovoltaic cell (ŋ) is given by the formula below.
Ŋ = Pm / (E * Ac)
The standard test conditions correspond to the spectrum and irradiance of sun rays incident on the solar panel tilted at 37 0, while the sun is at 41.81 0 above the horizon. In the United States, the approximation of this condition is experienced at solar noon close to the autumn and spring equinoxes when the solar cells face the sun directly. The above formula, therefore, would be very useful in determining the surface area of the panel or the number of cells required to produce a certain amount of power.
Solar modules, the rated power output is usually given by its maximum power (Pmax) in ratings and is calculated as the product of the maximum power voltage (Vpm) and current (Ipm); that is, Pmax = Vpm * Ipm. According to Solar Guide, “a 50 watt” solar module would “be about two
Square feet” or rather 1858.0608 square centimeters. Using this as the guideline to relate the size of solar panels (modules) to their power output, then we can determine the size of solar panels that produce power outputs of 85 kilowatts and 100 kilowatts.
The sizes of the photovoltaic module panels with the power output of 85 kilowatts and 100 kilowatts would be as follows; the calculations are based on the values above (a 50-watt solar panel would be 1858.0608 cm2). It is also worth noting that the power output of solar panels is dependent on their sizes and that 1 kilowatt (kW) is equal to 1000 watts (w).
- The size of the 85 kilowatt solar panel would be as below:
50 w = 1858.0608 cm2
85 kw = ( 85 * 1000 * 1858.0608 ) / 50 = 3,158,703.36 cm2
- The size of the 100 kw solar panel would be as below:
50 w = 1858.0608 cm2
100 kw = (100 * 1000 * 1858.0608 ) / 50 = 3158703.36 cm2 =3,716,121.6 cm2
Referring to the figures above, it is valid to deduce that one panel would be sufficient for areas that are 45 cm by 65 cm and 40 cm by 60 cm. Don’t forget that the maximum energy conversion achieved with thin-film-based panels is 17.2 %. Therefore, it is possible to get the amount of loose energy.
The amount of loose energy that is going to be from the panels assuming the average energy conversion of 17.2%, is as follows; since only 17.2 % of the total energy is converted to electrical energy, the rest 82.8 % is loose energy. Therefore, noting that both 85 kilowatts and 100 kilowatts = 17.2 % of the total energy supplied to the solar panel, 82.8 %, which is the loose energy for the two power outputs, would be as follow:
If 17.2 % = 85 kilowatt
82.8 % = (82.8 * 85) /17.2 = 409.1860 kilowatt
100% = (82.8 * 100) / 17.2 = 481.3953 kilowatt
These values are based on the most efficient thin-film-based solar panels, and various solar panels have varying efficiencies depending on the material constituting the solar cells. It is worth noting that loose energy is the amount of light energy that is not converted into electrical energy. It is expected all the light rays hitting the photovoltaic cells would be converted into electrical energy. However, this is not the case because of various factors that are dependent mainly on the material making the photovoltaic cells and the environment.
Therefore, thin-film photovoltaic cells have an efficiency of about 10%. Monocrystalline silicon cells have an efficiency of about 25%, while polycrystalline silicon has an efficiency of 20%. So the loose energy is either reflected or conducted as heat energy.
The above calculation is based on 250 Celsius, although they can tolerate temperatures that are above 400 Celsius. It is important to understand that the output of the solar panel would, therefore, be reduced as temperature increases.
How batteries reserve energy
Batteries store electrical energy obtained from solar panels in the form of chemical energy. A battery is made up of voltaic cells, each of which has two half-cells linked together in series by an electrolyte that has the ability to conduct electricity through anions and cations. The half cells include one, the anode (or the negative electrode) and the electrolyte, and two, the cathode (the positive electrode) and the electrolyte. The anions (or the ions with a negative charge) migrate to the anode, while cations (ions with a positive charge) migrate to the cathode. The charging and discharging of the battery is characterized by a redox reaction that involves the migration of anions and cations to the electrodes.
The process of storing electric energy into the battery as chemical energy, which is known as charging, involves reduction reaction ( or acquiring of electrons ) by the cations at the cathode while the anions at the anode undergo oxidation (or lose their electrons). During the oxidation reaction, the hydrogen atoms at the cathode free away to react with the electrolyte, while during reduction, the anode absorbs the hydrogen atoms. Basically, this process of electrochemical reactions involves removing electrons from the positive electrode and depositing them into the negative electrode. When the battery is full, this electrochemical reaction stops, and further charging will result in the electrolyte being converted into an insoluble substance; this is destroying the battery. The discharging of the battery, which occurs when the battery is powering a load such as electrical equipment, is the reverse of the charging process. It is worth noting that the electrodes remain separated and are only connected electrically by the electrolyte.
Types of batteries in the market
There are two main categories of electrochemical batteries; primary batteries and secondary batteries. In practical terms, the primary batteries only convert chemical energy into electrical energy without the reversible process. Therefore, after the exhaustion of the initial chemical reactants, it is not possible to restore the energy through the electrical process (Dingrando 2007). On the other hand, secondary batteries are rechargeable, meaning that their electrochemical reactions are reversible through the supply of electrical energy to the battery’s cells.
Electrochemical batteries are also generally distinguished by the type of electrochemical cells constituting them. These cells are of several types. They include voltaic pile cells, flow cells, galvanic cells, fuel cells, and electrolyte cells (www.pspb.org).
The electrolytic cells use electrical energy to decompose chemical substances through a process known as electrolysis. As a result, the chemical energy increases. Examples of the electrolytic cell include the lead-acid batteries and the nickel-metal hydride (NiMH) battery, categorized as such because of their behavior during charging. Nonetheless, the behavior of these two types of batteries – lead-acid and NiMH batteries – while they are discharging defines them as galvanic batteries.
The galvanic cells, which is sometimes referred to as voltaic cells, are electrochemical cells that are comprised of two half-cells (of different metal) connected together by a porous disk or a salt bridge. The lead-acid batteries are widely used rechargeable batteries and are commercially viable.
The other types of cells, such as the flow cells and the fuel cells, are applicable in various cases. Fuel cells are distinguished from electrochemical cells by their characteristic to use external reactants (Science Reference Services 2007); thus, they represent an open thermodynamic system as opposed to the closed system in electrochemical cells. Flow cells are a kind of rechargeable electrochemical cells.
Flow batteries are usually employed for comparatively large stationary applications. For instance, flow batteries are used in storing relatively inexpensive night-time electrical energy when electricity is cheap for use when electricity is more expensive, and for storing electrical energy generated from sources such as solar or wind energy, as well as for Uninterrupted Power Supply (UPS), which is used during main-power failures. Moreover, flow batteries are used in electric vehicles, and wind farm uses because of their ability to recharge rapidly.
Conclusion
The production of solar energy is relatively cheap. However, the initial installation cost of solar modules systems is very high. Moreover, on top of the maintenance costs of the battery and the fact that the devices used in the solar electrical system have a limited lifespan, the use of solar modules as the main source of electrical energy is still limited. However, with the ongoing research and discoveries in this area, solar modules systems may become viable and inexpensive alternatives to mains electricity.
References
“Spotlight on photovoltaics and fuel cells – A web-based study and comparison“, 2009. Web.
Dingrando, L 2007, Chemistry – matter and change, electrochemistry, Ch. 21, pp. 662–695, Glencoe/McGraw-Hill, New York.
Gibilisco, S 2006, Alternative Energy Demystified, McGraw-Hill Professional.
Hirshman, W, P, Hering, G, Schmela, M 2008, ” Market survey – cell and module production 2007 “, Photon International, pp. 140–174.
Sandia National Laboratories, The photovoltaic effect, 2009.
Science Reference Services 2007, Batteries, Super-capacitors, and Fuel Cells – Scope, 2009.
Creative commons 2002, Solar Cells: Shedding a little light on photovoltaic, 2009. Web.
Ramanathan, K, Teeter, G, Keane, J.C, Noufi, R 2005, “Properties of high-efficiency CuInGaSe2 thin film solar cells”, thin solid films, vol. 480 – 482, pp 499 – 502, National Center for Photovoltaics, United States
Sanyo Energy Corp. 2009, Think Gaia for life and the Earth: HIT photovoltaic module, 2009.
Solar Energy Alliance, Sanyo, 2009. Web.
The Solar Guide, Solar panels 2009. Web.