Abstract
Aerodynamics is the study of properties of fluids in motion and the manner in which they interact with solid objects. It explores the properties of solid objects as they travel through the fluids. Thus, aerodynamic principles are to explore the movement of aircraft in the air. The term supersonic refers to the movement of an object with speed higher than that of sound. Aircraft models designed to travel at speed greater than the speed of sound are called supersonic aircraft.
During the flight, the aircraft interacts with air particles to create pressure waves called the shock wave. The interaction between the air particles and the moving craft creates a thunder-like sound called the sonic boom. The transition between the sonic and supersonic regimes is called the transonic regime. As the aeroplane moves across the transonic region, shock waves are formed, and their movements have an impact on the flight. To overcome such challenges, a supersonic wing design must exhibit the ability to withstand wave drags. The SR-71 is an aircraft developed to travel at a supersonic speed.
Introduction
Aerodynamics is a branch of fluid mechanics dealing with the motion of air. It studies the interaction between air and solid objects such as aircraft. The main principles of aerodynamics used to describe aircraft movement are transonic, subsonic, hypersonic and supersonic (Carlson & Verberg, 2015). Supersonic is a concept used to study the properties of an aircraft moving at speed faster than that of sound. The speed is measured in Mach number, which is the ratio of the aircraft’s speed to the sound’s speed. When an aircraft moves at a greater speed than Mach 1, the motion is considered a supersonic flight (Graham, 2015).
Shock Wave
According to Graham (2015), a shock wave is the pressure wave propagating at a higher speed than that of sound in air. It is a nonlinear incidence experienced when rapid deposit and release of energy occurs in a confined region of the air. The unique aspect of the shock wave is that the wavefront where the compression takes place is prone to sudden and violent changes in temperature, stress, and density. Shock waves travel at a higher speed than sound and their speed increase with the increase in their amplitudes. However, their intensity decreases at a high rate because much of their energy is used to heat the medium in which they travel (Gerdroodbary, Jahanian & Mokhtari, 2015).
An aircraft travelling at subsonic speed generates pressure disturbances that propagate in all directions. Because these disturbances are continuously transmitted to the earth along the aeroplane’s path, they do not create sharp changes in pressure.
Nevertheless, the energy developed by the supersonic speed of the aircraft confines the pressure to a region that extends to the rear. In addition to the pressure from inside of the aircraft, they form a restricted cone called the Mach-cone. As the aeroplane moves, the cone’s parabolic edge creates a disturbance that intercepts the earth to produce a loud sound called the sonic boom (Gan & Zha, 2015). It also depends on the size and shape of the craft, atmospheric pressure, temperature and the movement of the wind (Carlson & Verberg, 2015).
As an aircraft moves up, transmits a force that creates a pressure change in air particles in front. Consequently, the air starts to move aside to prepare the way for the aeroplane to pass (Iuliano, Quagliarella, Donelli, Din, & Arnal, 2011). As the speed of the aeroplane approaches that of sound, the forward force increases and the change in pressure head can no longer warn the front air particles to pave the way for the approaching craft. What happens is that air particles accumulate in front of the aircraft and cause a sharp decrease in the flow of velocity with respect to the increased pressure and density (Gan & Zha, 2015).
As the speed of the craft increases beyond that of sound, the air in front of it is compressed. At some instances within the airstream, the particles of air are completely uninterrupted. In the next instance, the same particles are subjected to spontaneous changes in pressure, temperature, velocity and density (Graham, 2015). Consequently, shock waves are formed in the boundary between the uninterrupted air and the compressed air. Shock waves are created in the space between subsonic and supersonic areas during the transonic region (Gan & Zha, 2015).
A shock wave formed perpendicular to the airstream is called the normal shock wave. In this case, the flow of air that occurs immediately behind the wave has a subsonic speed. Airstream moving at supersonic speed and passes through normal shock waves will experience four changes. First, it will slow to the subsonic speed. Second, the airstream that forms behind the shock wave will maintain the same direction. Third, the airstream formed immediately after the shock wave will experience a great increase in static pressure and density. Lastly, the energy of the airstream will significantly reduce (Iuliano et al., 2011).
The formation of shock waves during a flight increases the drag. A dense high pressures area, which is unstable, forms immediately behind the waves. At the same time, the velocity energy within the airstream is converted into heat energy as it flows through the shock wave (Graham, 2015). These two phenomena increase the drag. However, the drag that results from the separation of airflow is greater than the initial one. When a strong shock wave is formed, the boundary layer does not have enough energy to endure the separation of airflow (Gan & Zha, 2015).
A significant increase in the thrust is necessary to increase the speed of a flight to the supersonic speed range. Depending on the angle of attack, design, and shape of the airfoil, the boundary layer formed earlier might reattach (Iuliano et al., 2011) as the normal shock wave develops on the upper surface of the wing, additional supersonic region forms. Moreover, a normal shock wave is created on the lower surface. The supersonic region enlarges as the speed of the flight approaches the Mach number. As a result, the shock waves move closer to the aircraft’s trailing edge (Graham, 2015).
Influence of Shock Waves in Transonic Region
The flow of air through a shock wave causes an abrupt increase in temperature, static pressure and density. On the other hand, this decreases the velocity of the air. An intense shock wave sufficiently reduces the velocity to separate the boundary layer from the surface of the aircraft (Iuliano et al., 2011). Such a situation causes instability in the airflow, which affects the transonic region in several ways.
During the subsonic flight, the deflection at the control surface causes pressure changes, which is felt in control itself and in front of the control hinge. The deflection of aileron affects the distribution of pressure from the head to the leading edge of the wing (Graham, 2015). However, changes in the pressure cannot propagate forward across the shock wave. When the formation of shock wave occurs on the aileron’s hinge line, the pressure changes resulting from the deflection is confined within the aileron’s surfaces. Consequently, the control forces generated by the aileron will reduce (Gerdroodbary et al., 2015).
When an intense shock wave occurs on the upper surface of the aircraft’s wings, the consequential boundary will reduce the lift being created. It will also cover the back surface of the aircraft in a turbulent airstream. The phenomenon directly reduces the elevators’ ability to produce control forces. As the aircraft accelerates from the subsonic to the supersonic region, the formation of shock waves and their backward movement causes the wings to have an aft movement (Gan & Zha, 2015). The backward movement and the loss of downwash above the tail’s plane cause the aircraft to pitch its nose downwards.
Sonic Boom
When an aircraft moves at supersonic speed, it produces a thunder-like sound that can be heard by people on the ground. That sound is called the sonic boom, and it results from reactions between air and supersonic aircraft. A travelling aircraft produces a strong force that pushes the molecules of air aside. The sonic boom is a kind of shock wave created by the source over a period and piles up together consistently. The accumulated wave causes an unusually strong force that produces the thunder-like sound audible on the ground (Gan & Zha, 2015).
As an aircraft travels in the atmosphere, it constantly produces waves analogous to water waves produced by a travelling ship. At speed exceeding that of sound, these waves combine to create shock waves that propagating forward from the source. At the supersonic speed, the above process continues, and the sonic boom is dropped along the path of the aircraft. (Gerdroodbary et al., 2015).
Supersonic Wing Designs and Example of Supersonic Aircraft
During the supersonic flight, the speed of air increases. The condition leads to an increase in dynamic pressures and the stick forces, which must be applied to propagate the control surfaces (Iuliano et al., 2011). In order to overcome these problems, an aircraft travelling at the supersonic speed employs specific design features. The wing plays a major role in the aircraft’s flight. The layout of the wings influences many aspects such as drag, movement, lift and performance. Therefore, it is essential to include the right parameters that define the horizontal and vertical aspects of the wings. These parameters are the sweep angle, the shape of the aerofoil, twist, aspect ratio, tip geometry and incidence angle among others (Carlson & Verberg, 2015).
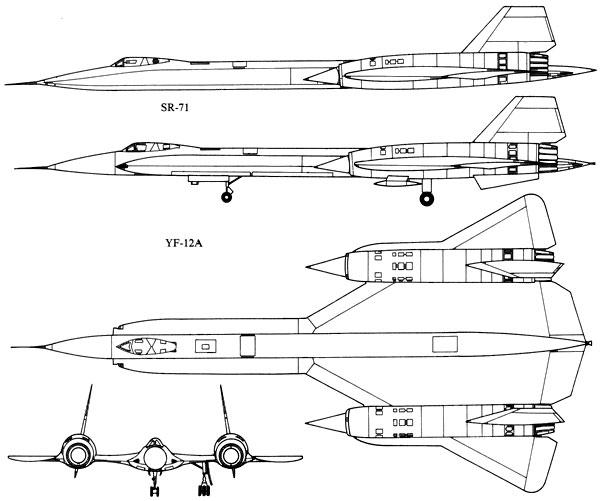
The design of a supersonic aircraft takes into consideration two issues. First, it must have the ability to fly in the subsonic range as it takes off and lands. Second, it must have optimized features to achieve the supersonic speed during the flight. Therefore, the design of a supersonic wing must have the necessary features to serve both subsonic and supersonic flights (Gerdroodbary et al., 2015). The geometry of the wings is important because it influences the aircraft’s aerodynamic properties. The main parameters of wings are a sweep, span aspect ratio, wing incidence, twist, wing dihedral, wing location and aspect ration (Carlson & Verberg, 2015).
The selection of the wingspan is the most important thing, which requires detailed study and evaluation. Sometimes, the size of the hangar and aircraft carrier might create a constraint in the span. A large span of these components, which is consistent with the dynamic constraints of the craft, reduces the drag (Gan & Zha, 2015). However, increased span contributes to the increase in the structural weight of the wing, which is essential in offsetting the drag.
It is important to stretch the span to achieve an optimum value (Iuliano et al., 2011). The sweep has a direct influence on the drag experienced within the transonic range. The correct choice of this component gives a higher value of the lift coefficient suitable for the supersonic design without divergence of the drag. What happens is that the sweep reduces the wave drag within transonic and supersonic regions.
The SR-71 is an example of a supersonic aircraft. It has a wing sweep of 600 that provides optimum performance during the supersonic flight (Graham, 2015). The sweep provides a greater angle of attack as it approaches the supersonic region and higher landing speed. The conceptual design can have the lowest chord ratio that gives an ideally thin plate. However, it is impossible to apply it in a practical situation because of the required structural strength. A thin plane is unable to withstand the force that propels an aircraft through the air. Having a high wing sweep helps in separating the horizontal tail and enhancing a delta configuration of the wing. The delta configuration of the wings allows for the combination of the elevator and ailerons to create elevons (Carlson & Verberg, 2015).
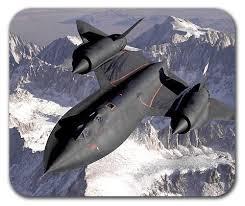
In addition, high wing sweep influences the design of the vertical tail, which can be compensated by a larger area. The SR-71 and other supersonic crafts use the twin tail combination to increase the surface of the vertical tail by two. The vertical fins help in creating additional yaw control during the flight. The vertical tail of a supersonic aircraft such as SR-71 has an impact on the movements of the yaw and the rolling motion. To reduce this impact, the aircraft has its vertical tail skewed inwards (Graham, 2015).
References
Carlson, A., & Verberg, R. (2015). A flight simulator for agile fighter aircraft and nonlinear aerodynamics. 53rd AIAA Aerospace Sciences Meeting. Web.
Gan, J., & Zha, G. (2015). Analysis of a low boom supersonic flying wing preliminary design. 53rd AIAA Aerospace Sciences Meeting. Web.
Gerdroodbary, B., Jahanian, O., & Mokhtari, M. (2015). Influence of the angle of incident shock wave on mixing of transverse hydrogen micro-jets in supersonic crossflow. International Journal of Hydrogen Energy, 40(30), 9590-9601.
Graham, H. (2015). The complete book of the SR-71 Blackbird: The illustrated profile of every aircraft, crew, and breakthrough of the world’s fastest stealth jet. Minneapolis, MN: Quarto Publishing Group USA Inc.
Iuliano, E., Quagliarella, D., Donelli, S., Din, S., & Arnal, D. (2011). Design of a supersonic natural laminar flow wing-body. Journal of Aircraft, 48(4), 1147-1162.
NASA Armstrong Fact Sheet: SR-71 Blackbird. (2014). Web.