Cell Theory
Cells are the basic unit of life that makes up every living organism. Cells were discovered by an English scientist known as Robert Hooke; he observed the structure of a thinly sliced cork under the light microscope which he invented (Khan Academy, 2015). He observed in the dead plant specimen small structures which he believed were ‘cells’. Schneiden and Schwann later observed the cells of an animal and concluded that all animals have cells that live and breathe. The last discovery was by Rudolf Virchow, where he discovered that all cells come from other cells. The cell theory has the following concepts; they only arise by division from an existing cell, they are the building block of all organisms, cells contain inherited information which controls their functioning, with favourable conditions cells can survive independently, and they undergo metabolism.
Eukaryotic and Prokaryotic Cells
There are two types of cells: eukaryotic and prokaryotic cells. Prokaryotic is the simplest and most ancient type of cells; they do not contain a nucleus, nor do they have any membrane-bound organelle. Prokaryotes can be grouped into two groups: bacteria and archaea. In contrast, eukaryotic cells contain a nucleus within every one of them, and their organelles are membrane-bound.
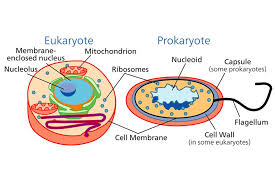
Differences between Eukaryotic and Prokaryotic.
Typical animal
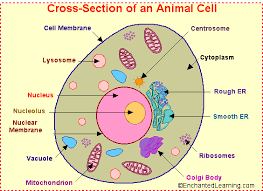
Animal cells are generally irregular in shape due to the absence of a cell wall. All animal cells comprise of the following organelles:
- Cytoplasm – Yellow fluid composed of mainly water. The cytoplasm is continuously streaming, and it is the biggest part of a cell. Organelles are suspended in the cytoplasm and salts are dissolved in it.
- Nucleus – It is the Control centre of the cell. It controls metabolism and reproduction activity of the cell. It contains the nucleolus which contains the genetic information of a cell.
- Endoplasmic reticulum – Endoplasmic lined with ribosomes is called rough endoplasmic reticulum while the one not lined is called the smooth endoplasmic reticulum. The endoplasmic reticulum is a system of membranous canals filled with fluid that carry materials around the cell. Ribosomes synthesize proteins.
- Golgi body – They temporarily store proteins which can then leave the cell via vesicles pinching off from the Golgi body.
- Lysosomes – Contain strong digestive enzymes which break down organelles as well as food.
- Mitochondrion – Organelle surrounded by a double membrane with the inner membrane being highly folded. Releases energy from the food inform of adenosine triphosphate.
- Vacuoles – Fluid-filled organisms enclosed by a membrane and can store food, water minerals and waste products.
- Cell membrane – Bi-lipid layer composed of protein and carbohydrate. The cell membrane separates the cell from its external environment, and it is selectively permeable. Selectively permeable meaning it controls what enters and what leaves.
Typical plant cell
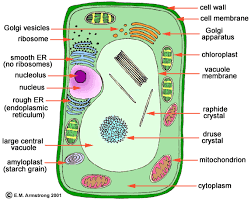
Plant cells are regular in shape and rigid because they have cell walls. Although plant and animal cells have some similar organelles with the same functions, there are those distinct to only plants these include:
- Cell wall – A rigid layer composed of cellulose polysaccharides and proteins which is found outside the cell membrane. The cell wall provides structural support to the cell and protects the cell from mechanical damage
- Chloroplast – It is an elongated organelle filled with the green colouring pigment in plants called chlorophyll which is required for the photosynthesis. The organelle also contain stroma which comprises of circular DNA
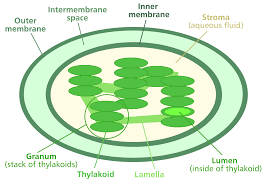
Microscopy
Microscopes are generally built to magnify, increase resolution and contrast of cells being observed under the microscope. The reason we use it on cells is that cells are too small to be seen by the naked eye. There two types of microscopy we are concentrating on, optical microscopy and electron microscopy. Optical microscopy uses light under a system of lenses to magnify samples which can be viewed directly by the eye.
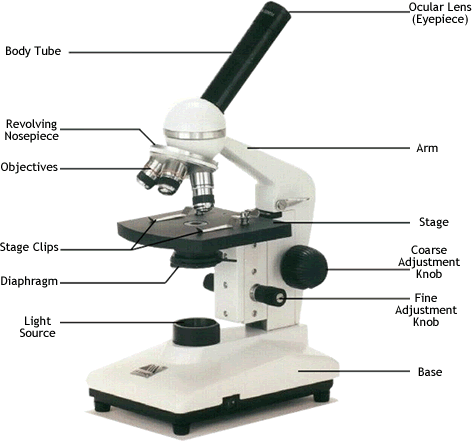
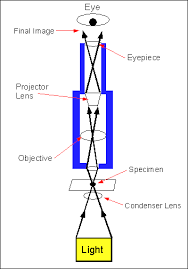
Light Microscope
A light microscope uses light rays that illuminate the specimen, making observation possible. The specimen is usually placed on a slide, stained with iodine and then covered with a slide and placed on the stage where clips secure it in position preventing the specimen from moving around. The light source produces light which passes through the condenser lens to the stage. The condenser lens mainly focuses light into a narrow beam to reach the specimen on the stage. On reaching the specimen, the light travels through the specimen to the objective lens, which magnifies the specimen. The objective lenses are usually four; hence the image can be viewed under different magnification factors. Light then travels to the eyepiece lens, which magnifies the image and converts the real enlarged image into an enlarged virtual image which is then projected to a receptor. The fine adjustment knob is turned to regulate the distance between the object and objective slightly to get the desired sharpness while the course adjustment knob which also moves the stage to regulate the distance between the specimen and the objective lens roughly and quickly
Magnification
Magnifications the size of an image divided by the size of an object
Magnification formula;
M= m1 ×m2
Where,
M=magnification
m1=linear magnification of the objective lens
m2=linear magnification of the eyepiece
Measuring a cell
Example 1
The image of a cell was measured to be 50mm, and the objective lens was set to *100.find the actual size of the cell.
Actual Size= Image Size / Magnification
50/100=0.5mm
Example 2
The actual size of a cell is 6 micrometers (µm), and the image of the cell was a measure to be 36 mm. Find magnifications?
Magnification = Image Size/Actual Size
1mm=1000µm therefore 36mm=36000µm
3600µm/6µm=×6000
Resolution
Resolution is the ability to distinguish between two distinct points. The greater the two points can be resolved, the greater the resolution.
Electron Microscope
This type of microscope uses electrons to view the image of the specimen. There are two types of electron microscope: The Transmission Electron Microscope (TEM) which allows electrons to pass through the specimen so one can see inside the cell and the Scanning Electron Microscope (SEM) collects the reflected and rebounded electrons off the specimen which gives the image of the outside surface.
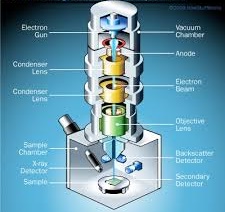
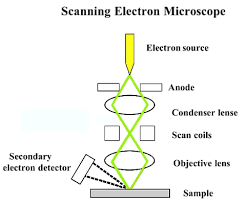
The electrons released from the electron gun form a beam by the help of the anode which brings the electrons together, preventing scattering. The electron microscope is usually filled by vacuum inside to prevent coalition of electrons with air particles. The electron beam then flows to the condenser lens whose function is to direct the electron beams onto the specimen which is placed on a grid. Before the sample, is the objective lenses which magnify the image. The beam then passes the specimen to the fluorescent screen below where the image is formed. The fluorescent screen glows wherever the beam of electrons hit.
Advantages and disadvantage of an electron microscope over a light microscope
The electron microscope has higher resolution capacity over a light microscope; the electron microscope does require tuning the focus like the light microscope it focuses at once on the image. The only weakness of the electron microscope is that the cell has to be dead.
Methods of preparing a Slide
Slide preparation depends on the samples structure; these methods include dry mount, wet mount, staining smear and squash.
- Dry Mount – The dry mount method can be used to observe dry specimens like pollen, hair, pollen and dead matter such as grasshoppers and aphids.
- Wet mount – Wet mounts are usually used for aquatic samples, living organisms which require liquid suspensions brine, glycerin and immersion oil. The slide is prepared by placing a drop of fluid on the slide, place the specimen on the slide, lower the cover slowly to avoid bubbles and remove water with a paper towel.
- Smear slides – The sample is usually liquid or slimes in this case. The sample is placed on a slide, and the second slide is used to smear the sample creating a thin layer. This is done slowly to prevent trapping of bubbles. The angle of the smearing slide determines the length of the smear.
A steep angle creates a shorter smear compared to a gentle angle.
Squash slides – This method is usually designed for soft samples. The slides are prepared by placing the tissue on a wet mount and press the cover glass gently to remove excess water. Caution not to damage the sample.
Plasma Membrane
The plasma membrane is phospholipid layer that surrounds the surfaces of cells and organelles. They are evident in both eukaryotic and prokaryotic cells. The plasma membrane has many functions. Its principal function is to acts as a barrier protecting components of the cells and organelles from the outside environment. The plasma membrane is composed of two phospholipids bilayer making it able to maintain the structure of the cells and organelles. The bilayer is formed due to the properties of phospholipids. A phospholipid has a hydrophilic phosphate head and two hydrophobic fatty acid tails. The phosphate head is said to be hydrophilic because it is attracted to water and the tails are said to be hydrophobic because they repel water.
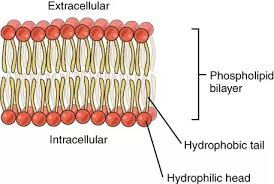
In an aqueous environment like the cells of our body, the phosphate heads position themselves to form the two outer rows because they are attracted to water molecules while the hydrophobic tails are protected by a non-aqueous core as a cluster to avoid the water. The plasma membrane is selectively permeable; it controls what goes in and out of the cell and organelles. Phospholipids membrane allows only oxygen, water and waste in and out of the cell but restricting entry of charged particles. The plasma membrane also contains other molecules that aid it in its functioning; these molecules include proteins, carbohydrates and cholesterol. Together the combination of these molecules form a fluid mosaic model.it is called the fluid mosaic model because the cell membrane is dynamic, the cell membrane moves on the soup, which is the phospholipid.
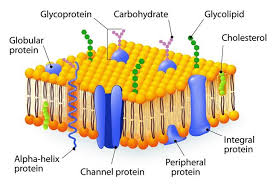
Membrane Proteins
There are several different proteins embedded in the membrane which serve a variety of function. They help with both facilitated diffusion and active transport. They connect cells; they participate in signal transduction and act as markers for cell identification. There are two types of membranes: intrinsic membranes and extrinsic membranes.
Integral Protein – Integral proteins are proteins permanently embedded in the phospholipid bilayer. They include carrier proteins which are used to transport molecules in and out of the membrane by active transport and facilitated diffusion, channel protein which allows facilitated diffusion. The channel protein provides passage for molecules that cannot travel through the phospholipid layer such as ions and water.
Extrinsic Membrane
These types of membranes do not interact with the hydrophobic core. A good example is glycoproteins. Glycoproteins are Short chains of carbohydrates are attached to proteins; they serve as recognition signals to other cells and also act as cell receptors. They sometimes receive growth hormones or other enzymes that signal the cell to do things
- Glycolipids – These are short chains of carbohydrates attached to membrane lipids; they offer cell recognition ability by other cells.
- Cholesterol – Cholesterol molecules are also found in the plasma membrane. They provide support and fluidity to the plasma membrane structure. They allow it to change shape, making it fluid.
Factors Affecting Plasma Membranes
- Temperature – Phospholipids are held together by hydrogen bonds that exist between the hydrophilic tails, them being held together tightly is what makes them semi-permeable. Hydrogen bonds are weak. When heat is applied, the molecules, they gain thermal energy and vibrate. The vibration causes the phospholipids to move more along the membrane and further apart from each other. The hydrogen bonds then break, causing the membrane to lose its structural integrity and the hydrophobic core to seize existing causing larger molecules to pass through easily. Besides, increasing the temperature also denatures the proteins. Denatured proteins can either prevent any particle from passing through or allow any particle to pass through depending on how they are denatured.
- Solvent type – Phospholipids have a hydrophobic core meaning they cannot allow any polar solvent to pass through them. However, when placed in a non-polar solvent, the solvent seeps between the tails and some of the solvent molecules get embedded within the membrane. The non-polar solvent molecules break the hydrogen bonds wherever they get embedded, making the membrane much more permeable.
Active Transport
This is the movement of particles against a concentration gradient from a low concentration gradient to a high concentration gradient by using adenosine triphosphate (ATP) involving protein carriers.
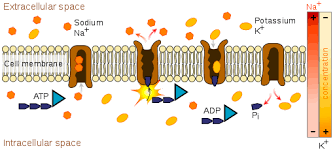
In order to move a particle from a region of low concentration to a region of high concentration, the protein carrier should have specific binding site for the particle. Different protein carriers have different shapes of binding sites depending on the shape of the particle. Once the particle is bound, adenosine triphosphate (ATP) binds with the protein carrier on the other side that faces the cytoplasm. The ATP is hydrolyzed into adenosine di-phosphate and a phosphate group to release energy. The energy is used to change the shape of the carrier protein so that the protein faces the side of the cytoplasm after which the particle is released to the region of high concentration. The phosphate group is thereafter released, allowing the carrier to go back to its normal shape since its chemical composition is reverted to normal.
Passive Transport
Living organisms have to be able to exchange substances such as food, oxygen and waste products between cells and their environment to survive. Passive transport can be defined as the transfer of substances across a cell membrane without requiring metabolic energy. The substances move across the membrane by the use of their kinetic energy. The two types of passive transport are diffusion and osmosis.
Diffusion
Diffusion can be defined as the net movement of particles from a region of high concentration to a region of low concentration across a partially permeable membrane. Specific particles are able to do this; these particles have to be small and non-polar to undergo diffusion easily.
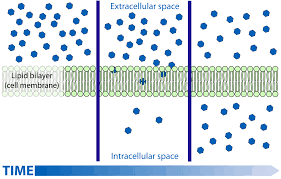
For diffusion to occur there has to be a concentration gradient. This means there has to be a region where there is more and a region where there is less. In regions of high concentration when particles move randomly, they collide. They move away from each other, and eventually, some of the particles will spread to regions of low concentration until they get evenly dispersed and reach equilibrium. At equilibrium, the particles still move freely because they still contain kinetic energy, but now there is no net movement but only equal movement in all directions.
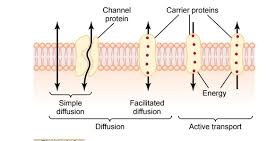
In cells when particles reach equilibrium, there is still passage of particles through the plasma membrane but instead of distributing themselves equally on one side, they move across to the membrane to the other region. At equilibrium, there is no net movement of particles.
Osmosis
Osmosis like diffusion is a passive process; it does not require any energy to occur as it depends on the constant random movement of molecules. Osmosis is defined as the net movement of water molecules from a region of higher water potential to a region of lower water potential across a partially permeable membrane.
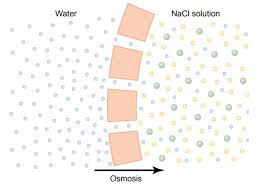
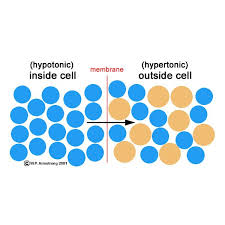
Water potential can be defined as the potential of water molecules to move out of a solution. The more a solute is added to water, the more its water potential drops. Similar to diffusion, the random movement of water molecules across the membrane will continue until they reach equilibrium.
Animal cells and plant cells are affected by osmosis. When animal cells are placed in a solution that that is less concentrated to their cytoplasm (hypotonic solution), water molecules will move across the cell membrane to the cytoplasm by osmosis. The cell swells and eventually may burst. When this process occurs in red blood cells, it is referred to as haemolysis. When the cells are placed in a solution that is more concentrated than their cytoplasm (hypertonic solution), Water molecules move out of the cytoplasm to the surrounding solution by osmosis. The cell shrivels. When this process occurs in red blood cell, it is referred to as crenation. When the cell is placed in a solution whose concentration matches the cytoplasm (isotonic solution) equilibrium is reached, and the cell experiences no structural change
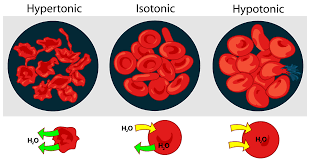
Due to the fact plants have a cell wall; they react differently from animal cells when placed under the same solutions. When plant cells are placed in a hypotonic solution, water molecules move into the cytoplasm, causing it to swell; hence the cell becomes turgid. The cell wall prevents the cell from bursting. Although, when placed in a hypertonic solution, water moves out of the cytoplasm by osmosis and the cytoplasm shrinks. The cell membranes move away from the cell wall; this process is referred to as plasmolysis. In an isotonic solution, there is no net movement of water molecules across the membrane causes the cell to be flaccid. Isotonic conditions cause plants to wilt.
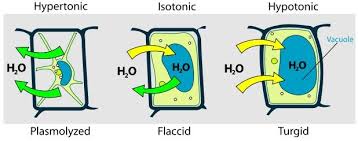
Exchange Surfaces
All living organisms require exchange to function at optimal conditions. Materials exchanged can vary from food, oxygen to waste products. In this instance, we are going to concentrate on gaseous exchange of mammals, fish and insects.
Insect gaseous exchange
Although insects are small, they are usually highly active, which means they require a constant supply of oxygen and removal of carbon (IV) oxide. An insect poses exoskeleton, which is a skeleton outside their body. The exoskeleton offers protection and water retention ability. Because of this exoskeleton gases in the air cannot easily diffuse into their cells, they bounce off. The carbon (IV) oxide that builds in their cell cannot diffuse out easily, causing a building up. They have evolved a specific type of exchange surface called the tracheal system, which delivers oxygen to every tissue and removes carbon (IV) oxide as well. The tracheal system has openings on the skin known as spiracles. The spiracles are openings that lead to larger airways in the system, which are called trachea. The trachea then divides into a smaller tube called tracheoles.
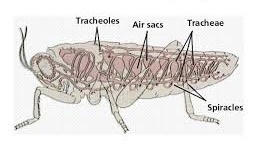
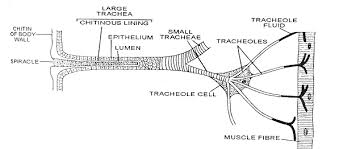
Gaseous exchange in insects usually occurs in fluid the end of the tracheal system called the tracheal fluid. This fluid exits at the end of the tracheoles. The tracheal fluid is in contact with the tissues and cells of the insect. When insects are active, their muscles require energy. The muscles draw up tracheal fluid containing oxygen-filled air away from the airways. The movement of the fluid away from the tracheoles also lowers pressure in the tracheal system drawing more air into the spiracles. This movement also increases the overall surface area of the tracheoles, making it easier for oxygen-filled air entering the tracheoles to diffuse into the tissues easily and carbon dioxide filled air out of the tissues. The oxygen taken in is then used by cells for respiration. When the insect is dormant, the fluid seeps into the tracheoles from the surrounding cells and lining the airways.
Ventilating the tracheal system
Ventilation is a specialized breathing mechanism that tends to occur in larger insects. This occurs when air sacs which are swellings in the airways are squeezed by flight muscles altering the volume of thorax pushing air in and out. Ventilation action continually draws in air with more oxygen and pushes out air with more carbon dioxide.
Other large insects have a different type of specialized breathing mechanism. It is specialized in how it works. As their abdomen is expanded by different muscle, this causes the spiracles in their abdomen to close and the spiracles in their thorax to open. Oxygen filled air enters through the thorax spiracles while those in the abdomen are kept closed. In the next cycle, the spiracles on the thorax close and those in the abdomen open, letting out the carbon dioxide filled air.
Fish gaseous exchange
Fish need to perform gaseous exchange just like any other animal. The only difference is that they do it underwater. Unlike land organisms, they take oxygen that has been dissolved in the water. This has made fish evolve specialized structure called gills so that they can exchange gases effectively. As they swim, water enters through their mouth and exit through the gills as they move. The gills consist of a series of bony arches with two stacks of gill filaments.
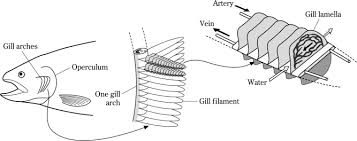
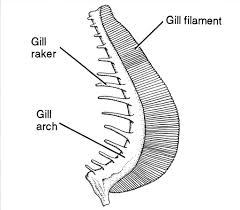
Gill filaments have protruding rows of very thin lamellae. Lamellae are used in biology to describe something thin and flat. Each lamella consists of a network of capillaries covered by a single layer of epithelial cells. Capillaries carry blood rich in carbon (IV) oxide (deoxygenated blood) from the body and as they reach the lamellae the branch into smaller capillaries. Capillaries are the site at which gaseous exchange occurs. After gaseous exchange, the capillaries re-connect and take oxygen-filled air (oxygenated blood) to where it is needed. The thin layer of epithelial cells provides a very small diffusion pathway. Being that these structures are very delicate, they are protected by a bony structure known as the operculum.
Bucall pumping
Buccal pumping is the method fish use to ventilate their gills by making freshwater move through the gills and deliver dissolved gases to those capillaries. The purpose of this is to maintain a strong diffusion gradient so that efficient gaseous exchange can take place. To ventilate the gills, they use the coordinated opening of the opercular vents with the closing of the buccal cavity. The buccal cavity means the fish’s mouth.
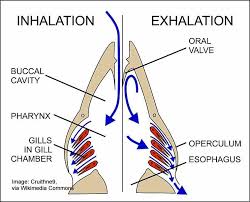
When the buccal cavity opens the opercular vents close and water is drawn into the buccal cavity which is then pumped over the gills providing oxygenated water to the gills. When the mouth is closed, the opercular vents become open and provides the pathway of water in the mouth to flow over the gills and escape out the vents taking deoxygenated water with it. Fish such as sharks do not have the ability of buccal pumping; therefore, they always need to swim to keep a fresh supply of water over their gills.
Counter current flow mechanism in the gills
The gill filament and the lamellae are oriented in such a way that they ensure water passes over them counter current to the flow of the blood in the capillaries. Counter-current can be defined as two liquids in proximity to each other flowing in the opposite direction. The counter-current flow ensures optimum gaseous exchange.
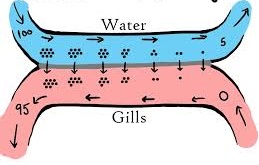
When water flows past the capillaries, it loses oxygen to the blood down the diffusion gradient. The oxygen in the water diffuses into the blood with low oxygen levels.
As the blood continues to flow, it continues to take in more oxygen from the freshwater entering the system. There are two steps in this: there is receiving oxygen from partially oxygenated water, and there is receiving more oxygen from freshwater entering the system. This maximizes efficiency
Mammal Gaseous Exchange
Mammals are large organisms; therefore, they require a large intake of oxygen; they also produce large amounts of carbon (IV) oxide. Therefore mammals evolved a specialized gaseous exchange system to satisfy the need for this requirement. They evolved the lungs which are generally found in the thorax. The lungs are encased in the protection of a group of ribs which make up the rib cage. The gaseous system is separated from the abdomen by a diaphragm. The diaphragm is flexible cartilage. Lungs are two in number, one for each side of the body.
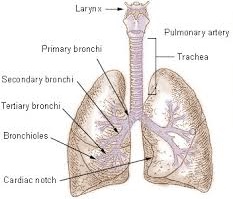
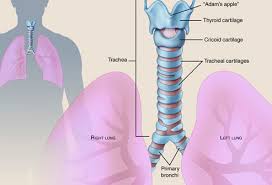
The lungs are connected to the mouth and nose by the trachea and bronchi. The mouth and nose are the portals of entry and exit for these gases. When mammals breathe in, air travels down the oral cavity and descends down the trachea. The trachea then divides into two primary tubes named the bronchi (bronchus in singular). These tubes are lined down with C-shaped cartilage rings. Cartilages are stiff tissues that provide support and allow little flexibility to the tubes. They provide support by keeping the airway open. The inner walls of the tubes are lined with ciliated epithelial tissue; ciliated cells are basically cells with hair at the top. The function of the ciliated cells is to waft mucus, dirt and pathogens in a particular direction. The walls are also lined with smooth muscles which contract and relax adjusting the diameter of the tube regulating the volume of air flowing in the tubes. The epithelium tissues also contain goblet cells which produce mucus that traps dust
The bronchi divide into smaller and smaller branches as they go deeper into the lungs. These branches are called bronchioles. They lead down to the alveoli, which are the smallest unit. They mainly consist of smooth muscles and some epithelial cells. The lungs have two-process cycle ventilation; inspiration and expiration.
Inspiration
Inspiration is the net movement of air in the lungs. This process is defined as active because muscles use energy to contract. The external intercostal muscles contract and the internal intercostal muscles relax. The diaphragm muscles contracts and moves downwards. Due to this movement, the ribcage moves outwards and upwards. The volume of the thoracic cavity increases, decreasing the pressure. The pressure difference between the external environment and the lungs causes air to move into the lungs, causing them to expand. Air flows to the alveoli where gaseous exchange takes place.
Expiration
Expiration is the net movement of air out of the lungs. This process is defined to be passive because it doesn’t require energy. The external intercostal muscles relax, and the internal intercostal muscle contract. The diaphragm muscle relaxes and assumes its normal dome shape. The ribcage moves downwards and inwards. Volume in the thoracic cavity reduces, and the pressure increases. The difference between the air pressure in the lungs and the external environment causes air to move out of the lungs.
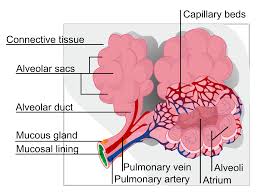
The lungs consist of millions of alveoli. The alveoli are lined with an extensive network of capillaries which provide a large surface area for gaseous exchange. The alveoli are covered with alveolar epithelium which is one-cell thick, thus providing a short diffusion pathway.
.
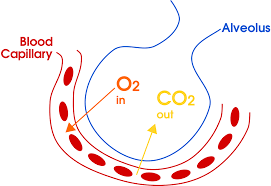
When we inhale all the alveoli expand and are filled with air, the oxygen-rich air dissolves in the moist layer and diffuses in the across the epithelial layer into the deoxygenated blood. At the same time, carbon IV oxide diffuses out of the capillary across the epithelial layer into the alveoli, which is then breathed out as carbon (IV) oxide rich air.
Transport in Plants
Transportation of food and water in plants occurs mainly in the vascular bundle. The vascular bundle consists of xylem and phloem.
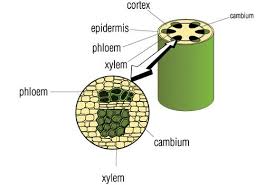
Xylem
Structure of the xylem
Xylem is continuous hollow tubes running throughout plants transporting water and minerals. The movement of water and minerals up the xylem is referred to as the transpiration stream.
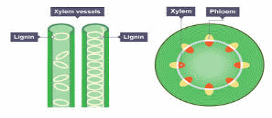
Xylem vessels are dead cells stacked end to end that form a tube structure that runs along the plant’s length. The xylem vessels have no cytoplasm or organelles. In addition, the xylem are lined with a waterproof polysaccharide known as lignin. The lignin coating prevents water from seeping out of the cell walls and supports the xylem.
Formation of xylem vessels
Through time cells age and begin to die. Different enzymes in the cell start breaking down the organelles in primary products. The cell wall at the top and bottom degrade while those at the side remain intact. After the cytoplasm and organelles have disappeared, lignin is deposited in a ring-like or spiral manner around the cell’s inner walls, making it waterproof, and ensuring free flow of water. Lignification is the process lignin is deposited. Some holes existing in the xylem that are not lignified they are referred to as bordered pits. These bordered pits allow water to move laterally from one vessel to another. The xylem vessels are made narrow enough to allow water to move upwards in a continuous flow.
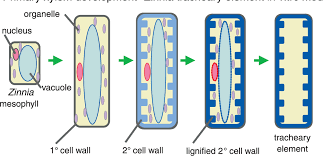
Phloem
When leaves synthesis sucrose from reactants of photosynthesis, the sucrose is transported by the phloem.
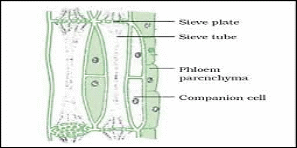
The phloem tissue consists of two types of cells: the sieve tube elements and companion cells. The sieve tube elements are stacked end to end, which allows the flow of sap. Sieve tube cells have little cytoplasm and no nucleus; this provides space for sap transportation. When two ends of two sieve tubes meet sieve plate forms. The sieve plate allows specific sized molecules to pass, and this is how the sap transports itself through these sieve plates. The other type of cell is the companion cell. These companion cells’ function is to control the transport of sugar and organic molecules in the phloem. Since the phloem requires active transport to move the sap in translocation, they are packed with mitochondria, which produce energy for active transportation. To provide support to the phloem is filled with parenchyma tissue.
Translocation
Translocation is the transport or movement of sugars and assimilates around the plants. After photosynthesis, the glucose converts into sucrose for transportation. This prevents the sugar from broken down easily by cells. For sucrose and assimilates to be transported, they first have to be loaded to the phloem by active loading. Active loading is the process of loading sucrose into the sieve elements. The process is as explained below.
In the companion cells there is a high concentration of hydrogen ions compared to that of the leaf tissue. Specific proteins existing in the cell membrane actively transport these ions into the leaf tissue, creating a diffusion gradient across the cell membrane. As a result, there will be fewer hydrogen ions in the companion cell than in the leaf tissue. After pumping out of hydrogen ions, they diffuse back into the companion cell. They do this through a transporter protein, which also takes the sucrose molecules back to the companion cell in the process. A higher concentration of sucrose in the companion cells increases the diffusion gradient against adjacent sieve tube elements causing sucrose to diffuse into them. They then transport the sugar around the plant.
Transport of water
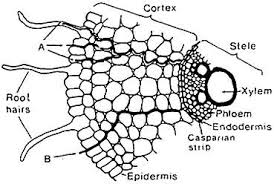
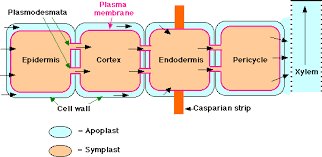
Water is absorbed by the root hair and transported to the xylem by water potential manipulation. Water moves in the root hair cell using two pathways; the apoplast pathway (through cell wall by water tension) and the simplest pathway (by osmosis through the cytoplasm). The root hair cells are also packed with mitochondria to facilitate absorption of minerals. For plants to absorb water, their cells have a higher negative water potential than the soil solution. This is achieved by actively transporting mineral ions from the soil. Highly packed mitochondria provide energy for active transport. Now that the cytoplasm has more negative water potential, water moves into the root hair cell by osmosis. The cytoplasm of the root hair cell is diluted. Adjacent cortex cells having more negative water potential than the cytoplasm; water moves into them by osmosis. This process repeats itself until the water reaches the endodermis, whose cell wall is coated with a Casparian strip. The Casparian strip is lined with waxy suberin making it waterproof. Water movement using the apoplast pathway halts, and water diverts into the symplast pathway. This transaction filters out any toxins in the water. Water potential manipulation, similar to that in the root hair cell, water moves from the endodermis to the xylem. Movement of water in the xylem generates root pressure, which, combined with transpiration effects, pushes the water up the xylem.
Transpiration
Transpiration is the loss of water vapor by evaporation through the stomata. Transpiration has some major benefits to plants: it helps plants in cooling by absorbing the heat and losing it to the environment and causes the transpiration stream. The transpiration stream is the movement of water up the stem using cohesion and adhesion forces. In contrast, transpiration has a disadvantage; when plant cells lose water, they become flaccid, leading to wilting.
There are three types of transpiration; lenticular transpiration, stomatal transpiration, and cuticular transpiration
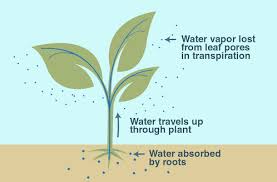
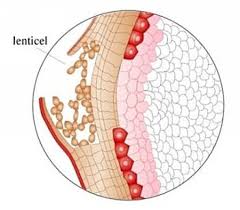
Stomatal transpiration is the loss of water vapor through the stomata. Stomata (stoma) are tiny openings on green plants where gaseous exchange takes place. Guard cells regulate the opening and closing of the stomata. The guard cells take in water from adjacent xylem tissue since they have more negative potential vacuole. The guard cell becomes turgid and causes the stomata to change shape, creating an opening through which water vapor escapes.
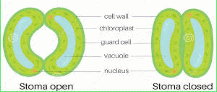
Factors Affecting Transpiration Rate
Transpiration is directly affected by the external environment. These include:
- Temperature – When temperature increases transpiration rate increases, and when temperature decreases transpiration rate decreases. Increased thermal energy increases the kinetic energy of water molecules; this increases the amount of water vapor moving out of the stomata.
- Humidity – When the humidity is high transpiration rate reduces and vice versa. Humidity affects the water vapor concentration gradient. The steeper the concentration gradient, the higher the transpiration rate.
- Wind – High wind speed increases the transpiration rate.
- Light intensity – Light intensity affects the opening and closing of stomata. When light intensity is high, the rate of transpiration increases, and when low, vice versa. Light intensity increases the rate of photosynthesis, causing the stomata to open. The space provides more passage for water vapor to exit.
Measuring the rate of transpiration
The rate of transpiration can be estimated using a photometer, as in the image below. The rate of transpiration is estimated by the air bubble in the capillary tube. Increased transpiration causes the bubble to move to closer to the reservoir tank, and when it reduces, the bubble moves away.
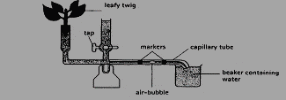
Terrestrial Plant
Differences in climatic and topographical affect how plants live. For plants in extreme conditions to survive, they develop adaptations that help them regulate activities. Plants in dry conditions and plants in extremely wet conditions are referred to as xerophytes and hydrophytes, respectively.
Xerophytes
These are plants that adapt to live in areas where their water loss due to transpiration may exceed their water uptake. These adaptations enable them to retain water. Xerophytes have a thick waxy cuticle, which is waterproof. The cuticle reduces water loss by evaporation from epidermal cells. Some plants have curly leaves that protect their stomata from exposure. The curl helps trap a region of still cold air, reducing the transpiration gradient, reducing water loss. Xerophytes also have hairs near their stomata, which traps moisture. This lowers the water potential gradient between inside the leaf and outside, reducing transpiration. In addition, xerophytes have reduced leaves, which reduce the surface area of water loss. Lastly, some xerophytes have thick stems that store water.
Hydrophytes
These are plants that adapt to living in areas where water gain is excessive and may exceed water loss. Hydrophytes living on water have lots of aerenchyma tissue that fill up with air making them buoyant. They also have a thin layer of waxy cuticle, increasing the evaporation rate in the top epidermal cells. Unlike xerophytes, they have more stomata on their leaves, increasing the transpiration rate and allowing the plants to access gases.in addition, hydrophytes have poorly developed roots or no roots.
Biodiversity
Biodiversity is the measure of the variety of species in an area (species richness). Species tend to cluster in particular areas across the world, so different groups of living organisms live in specific habitats. For instance, giraffes are generally found in Africa while pandas in northern Asia. A species is a group of organisms which are capable of interbreeding and produce fertile offspring while a habitat is where an organism lives. In an ecosystem, there are different types of species co-existing with one another, and this forms areas of biodiversity.
There are three levels of biodiversity; species, genetic and ecosystem diversity. Species diversity refers to the number of species and the number of their individuals in a specific area. Genetic diversity is a measure of genes possessed by individuals in a population or whole species. Genetic diversity comes about when individuals within a species with different genetic structure produce a fertile offspring. The causes of genetic biodiversity include mutation and cross-breeding. Populations with higher genetic diversity tend to be healthier than the population with low genetic diversity. Ecosystem diversity is the measure of the range of habitats on arrange of scale from a small ecosystem to the whole world.
Sampling
Before calculating biodiversity, we need first to know the number of all these different species. This is done by sampling. There are two types of sampling; random sampling and non-random sampling. Before going to the field, biologists are required to have; proper clothing depending on which habitat they are going to sample, recording materials, equipment’s for sampling and classification keys to help classify organisms.
Random sampling
Random sampling is one of the easiest methods of collecting data. In random sampling, there is an equal probability of selecting each species from the population studied. The most common method used is quadrant sampling. The quadrant used can be of any length and width. The area of the quadrant is then split into a (1×1) square grid. Each square grid is assigned coordinates. The coordinates to be sampled are randomly chosen. 5%-10% of the total area should be sampled for accuracy. Chosen organisms in the selected square grids are then counted. For organisms that are at the border of the quadrant, more than50% of their body needs to be in the grid to be counted; otherwise, they are left out. After recording the data, a mean average of the organisms per grid is calculated. To estimate the total population, the mean average is multiplied with the total number of (1×1) square grids.
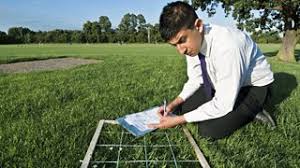
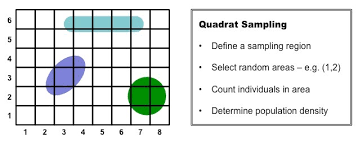
Example
In a school football field daisies grow randomly on the ground. Find their population density using random quadrant method.
Football field area= 620m2
Area divided into (1×1)m2 grid boxes
Daisies in 9 grid boxes were counted and recorded as below
Mean average of daisies/ m2 = 7
Total population density (7×620)= 4,340 daisies
Non –random sampling
There are three types of non-random sampling; opportunistic, stratified and systematic sample. The opportunistic method is when the area is chosen by the researcher using sight or prior knowledge.the method has more bias, larger species may be noticed faster than smaller species. The stratified method is when the area is divided into groups which are sampled differently. The opportunistic method prevents small population from being missed out. Finally, the systematic method is when samples are taken at set intervals between each other. This can be done along a tape measure. As only small areas are sampled there is a risk of missing species which underestimates the biodiversity of the area.
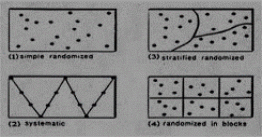
Calculation of Biodiversity
This is done using the index of diversity, which is a measure of species diversity. It considers the number of species in a community and the number of individuals in each species. Index of diversity defers from species richness which is the number of species in a community. Calculations are done using a formula called Simpson’s index of biodiversity.

Example
Biologist collected the data below from a home garden in the amazon. Using the data in the table calculate the biodiversity in the garden using the Simpson’s index?
SDI=100(100-1) /2900
SDI=3.1
Factors affecting biodiversity
There are three main influences of biodiversity; human population growth, agriculture and climate change.
Human population growth
An ever-increasing human population inevitably results in deforestation for the building of houses and roads; this destroys the habitats of organism reducing biodiversity. The increasing human population also results in more waste products, and toxic gases polluting the environment. Pollution kills organism decreasing biodiversity in certain areas.
Agriculture
In order to feed an ever-growing population of humans, large areas of land are devoted to monoculture. Monoculture is the mass growing of a single variety of a crop leading to a reduction in biodiversity for the following reasons; large areas of land are cleared for planting crops ruining natural habitats of organisms, herbicides are regularly used in agriculture killing many species of plants and animals. Local plants and animals are seen as weeds and are exterminated, reducing biodiversity. Lastly, monoculture reduces certain nutrients in the soil, making the soil unconditional for the growth of certain plants.
Climate Change
Climate change is one of the most significant challenges facing humans. Climate change refers to changes in average weather conditions such as rainfall, temperature and wind in a region over a long period and affects biodiversity in this various ways; rising temperature may cause some animals to migrate or become extinct because they are physiologically unable to handle such conditions. This lowers biodiversity in the region. Rising temperature also causes the polar ice caps to melt, which causes flooding by rising sea levels. Flooding destroys habitats reducing biodiversity in flooded areas but also increases biodiversity for the sea organisms. Change in rainfall also affects biodiversity; it increases biodiversity in others while reducing biodiversity in others.
Maintaining biodiversity
Humans have devised new techniques to rectify the mistakes they have made, and these include; practicing organic farming which is the use of organic manure rather than fertilizers. This farming tends to use less machinery to reduce pollution and rotate their crops to prevent over usage of specific nutrients in the soil. Humans have also resorted into renewable energy such as hydroelectric power and solar power, reducing pollution, which in turn reverse climate change. Afforestation and reforestation are practiced in specific areas in the word to increase habitats for organisms increasing biodiversity.
Biological Molecules
There are four main types of biological molecules found in all living organisms. These are carbohydrates, proteins, lipids, and nucleic acids. They are made up of carbon and hydrogen atoms and contain a small number of other chemical elements. They usually have a large number of atoms, so they are also known as macromolecules. A lot of biological molecules are polymers which are built up from repeating smaller units called monomers.
Condensation and hydrolysis reaction
Monomers combine to form polymers by a condensation reaction. A condensation reaction is a reaction that occurs when two molecules combine to create a more complex molecule with the removal of water. Water is formed by coming together of hydroxyl from one monomer and hydrogen from another monomer.
A polymer is broken down into monomers by hydrolysis reaction. Hydrolysis requires water in order to break the bond between the monomers. Therefore, it is a reaction that occurs when large molecules are broken down into smaller molecules by adding water.
These reactions are used to build up or break down biological macromolecules.
Carbohydrates
Carbohydrates are the most abundant of the biological molecule. They are useful in many different ways. This includes:
- They store energy in our body in the form of glycogen
- They form the cell wall of plant cells that give the cells their rigidity
They contain carbon, hydrogen, and oxygen atoms.
In carbohydrates, the monomers are called monosaccharide and the polymers are referred to us as polysaccharides.
Monosaccharide
They are the simplest carbohydrates and so they form the building blocks for more complex carbohydrates.
Their general formula is;
(CH2O)n, where n is any number of three or greater.
Some of their physical properties are;
- They are soluble in polar solvents such as water.
- They are sweet tasting and that is why they are also called sugars.
- They can form crystals.
A monosaccharide can either be a triose, tetrose, pentose, or hexose based on the number of carbon atoms. The general formula is used to work out the molecular formula of each type of monosaccharide. The six-carbon sugars, hexose, are the most common, for example, glucose
Glucose can exist as an isomer. Isomers are molecules with the same chemical formula but different arrangements of atoms in space. It has two isomers: beta and alpha glucose. They differ in the position of the hydroxyl group at carbon 1
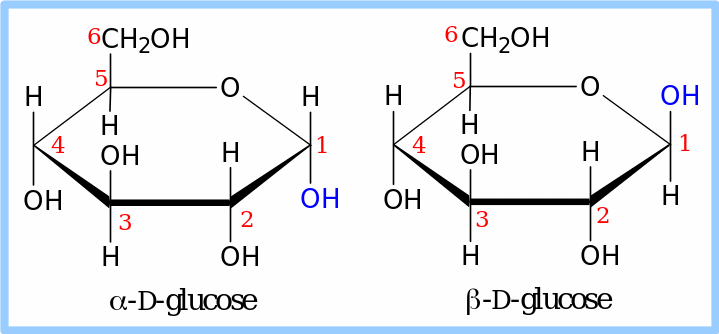
Thus, different polysaccharides are formed depending on whether alpha glucose or beta glucose is used as a building block—for example, starch- alpha glucose, cellulose -beta glucose.
Disaccharides
When one monosaccharide combines with another through a condensation reaction, they form a disaccharide. Just like the monosaccharide, disaccharides are sugars and soluble in water. Different combinations of monosaccharides determine which disaccharide is formed.
Glucose + Glucose = Maltose
It is found in germinating seeds
Glucose + Fructose= Sucrose
It is common table sugar.
Glucose + Galactose= Lactose
It is found in mammalian milk
In the condensation reaction of carbohydrates, a glycosidic bond is formed between the monomers. This is a covalent bond that forms by a condensation reaction in carbohydrates. Glycosidic bonds are named depending on which carbon atom they are formed in between.
Polysaccharides
Polysaccharides are formed when more than ten monosaccharides join together, although there are also chains with less than ten polysaccharides. These chains are called oligosaccharides. They are insoluble in water and so they are not sugars. Examples of polysaccharides are starch, glycogen, and cellulose. Storage polysaccharides such as starch and glycogen can store energy because:
- They are readily hydrolyzed to glucose when energy is needed.
- They are compact, so a lot of energy can be stored in a small space
- They are large so that they do not diffuse out of the cell.
- They are insoluble in water, so they do not affect the osmotic balance of the cell.
Starch
It is a storage polysaccharide in plants. It is formed from alpha glucose molecules forming 1, 4 and 1, 6 glycosidic bonds. Starch particularly numerous in photosynthesizing cells in leaves and in storage organs such as tubers and seeds. Glucose is stored in the form of amylose and amylopectin, which are collectively known as starch. Amylose is a long chain of alpha glucose polymer joined by 1, 4 linkages. It coils up into a helix shape to make it more compact. It has only two open ends for the amylase enzyme to bind; hence it is broken down slowly, making it not the best way to get alpha glucose from an energy store.
Amylopectin is also a long chain of alpha glucose polymer joined by 1, 4 glycosidic linkages but unlike amylose, it has occasional 1, 6 glycosidic bonds that make it branched with more accessible ends for enzymatic action
Glycogen
It is a storage polysaccharide in animals and bacterial cells. It is highly found in cells with high metabolic rates such as liver cells and muscle cells.it is similar to amylopectin but glycogen has more 1, 6 glycosidic linkages bringing about a highly branched structure. This means it has more accessible ends for enzymes during hydrolysis; consequently, more energy is released to satisfy the higher metabolic requirement in animals.
Cellulose
It is a structural polysaccharide found in the cell wall of plant cells. It is formed by beta glucose monomers and has 1, 4 glycosidic bonds. They are straight parallel chains with no branches. Hydrogen bond forms between parallel chains forming microfibrils which then form fibers. This makes cellulose very strong.
Humans lack the cellulase enzyme needed to break the 1, 4 linkages between beta glucose molecules. However, they need cellulose as it provides the fiber necessary to keep their body healthy.
Proteins
Proteins are organic molecules that have many important functions, including:
- Structural role- build up some structures of the body, say muscles and tendons
- Metabolic role- enzymes are proteins involved in metabolic reactions
- Transport role- hemoglobin is a protein that transports oxygen in the blood
Carbon, hydrogen, oxygen, nitrogen, and occasionally sulphur or phosphorus make up the structure of proteins.
Amino acids are the monomers, while polypeptides are the polymers.
Amino Acids
They are the building block of proteins. There are 20 types of amino acids but they all have the same basic structure. They have a central carbon(C) atom bonded to a carboxyl group (-COOH), an amino group (-NH2), a hydrogen group (-H), and a variable group (-R).
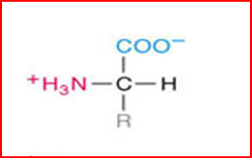
Each amino acid has a different R group that can vary in size, polarity, and charge (acidic and basic). This gives every amino acid different properties. When an amino acid joins with another amino acid through a condensation reaction, they form a dipeptide. Many amino acids joined together to form a polypeptide, a protein polymer. A protein is formed when one or more polypeptides fold into a specific shape that allows it to perform its precise function. The condensation reaction occurs between an amino group and a carboxyl group on the adjacent amino acid. A peptide bond is then formed between the amino acids and is commonly referred to as a dipeptide bond.
Protein Structure
- Primary structure – This is the linear sequence of amino acids in space. It is coded for by DNA and determines the entire series. There is an almost limitless combination allowing every protein to have a unique primary structure.
- Secondary structure -Hydrogen bonds form between amino acids; as a result, the chain folds into two primary shapes:
- Beta pleated sheets- the chain form a zigzag and fold over themselves.
- Alpha helices- the chain coils with hydrogen bonds keeping the coil stable
Although hydrogen bonds are usually weak, there are many hydrogen bonds in the secondary structure, stabilizing the entire structure.
- Tertiary structure – A protein with a 3D structure is formed after the alpha helices and beta-pleated sheets randomly coil and twist with each other. Three types of bonds are involved that form between the R groups of the amino acid: di-sulphide bridge, hydrogen bonds, and ionic bonds.
- Quaternary structure – Many polypeptides aggregated with prosthetic groups (non-protein), for example, hemoglobin
Shape of Proteins
This is split into two main groups.
- Globular proteins for metabolic functions. They are round
- Fibrous proteins for structural functions. They are straight
Lipids
Unlike other organic molecules, they are not polymers. They come in two main types: triglycerides and phospholipids. They are mostly made up of carbon, hydrogen, and oxygen. They are nonpolar molecules and therefore, they are insoluble in water but soluble in organic solvents.
- Triglycerides – They are created from one molecule of glycerol and three molecules of fatty acids. They are a good source of energy.
- Phospholipids – They are formed from one molecule of glycerol, two fatty acids molecules, and a phosphate molecule. They are structural lipids that form the cell membranes.
Functions of Lipids
- Are used as structural components in the cell membrane
- Some serve as insulators between the cells and the environment.
- They are also used as essential sources of energy-rich foods and cooking oils.
- Are shock absorbers-prevent organs from mechanical injury
Test for Lipids
Emulsion test. A cloudy white emulsion forms if lipids are present but remain clear if not present.
Transport in Animals
Animal Transport System
Multicellular organisms have a complex transport system known as the circulatory system and it comprises a network of blood vessels, specialized organs, and the blood.
A good transport system should has:
- Exchange surfaces that enable substances to enter the fluid e.g., Capillaries
- A pump to create pressure for the circulation of the transport fluid
- A fluid medium to carry substances e.g., blood
Circulatory systems
An open circulatory system is one in which blood flows freely through the body cavity as in insects. A closed circulatory system is where blood is contained in blood vessels and also involves a heart that pushes the blood around the body, such as in fish and mammals.
A single circulatory system- the blood flows through the heart once for each circuit of the body. (imperiya.by, 2019) For instance: in fish. A double circulatory system is where blood flows through the heart twice for each circuit of the body, like in mammals.
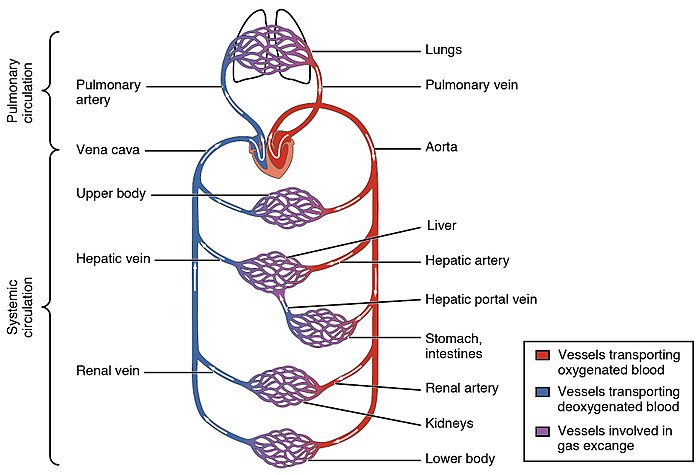
Blood Vessels
Transporting blood in vessels allows it to be pumped at high pressure delivering nutrients and removing waste more efficiently. The circulatory system has five different types of vessels: arteries, veins, arteriole, venules, and capillaries. These vessels are adapted slightly differently to different roles based on how far away they from the heart. But all the blood vessels have a feature in common. They have a smooth and thin inner layer of cells called the endothelium.
Arteries
Blood is carried from the heart to the rest of the body through the arteries. It is carried in very high pressure so that it can reach farther parts of the body. The artery walls are very thick to cope with the high pressure since they have thick layers of elastic tissue and smooth muscle encased in a thick outer layer of collagen. The collagen provides the necessary strength, while elastic tissue enables it to stretch and recoil to maintain the high pressure. Smooth muscles contract and constrict the artery narrowing the lumen. The lumen is narrow to keep the pressure high.
Arterioles
They carry blood from the arteries to the capillaries. Arterioles have a similar structure to arteries, but they are smaller and have relatively thinner muscle and elastic layers. The contraction of smooth muscle constricts the arteriole and is used to control the blood flow through the body by diverting blood to regions that need more oxygen.
Capillaries
Capillaries are thin-walled vessels consisting of just the one cell thick endothelium. The lumen of capillaries is very narrow and can squeeze red blood cells against the endothelium to reduce the diffusion distance of oxygen. It has small gaps on its wall to allow dissolved substances and water to leave and enter the blood.
Veins
Veins carry low-pressure blood back to the heart. Veins have thin walls and wide lumens to allow a lot of deoxygenated blood to return to the heart. Veins have valves that prevent the backflow of the low-pressure blood, ensuring it flows in the right direction. Movement of skeletal muscles in the body compresses the veins and pushes the blood along.
Venules
Venules are smaller than veins and lack valves. They carry blood from the capillary beds to the veins and consist of a thin layer of muscle and elastic fiber.
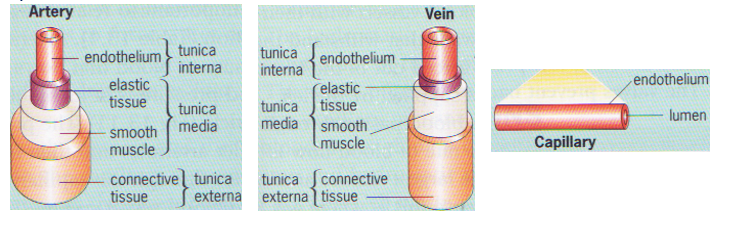
Tissue Fluid
This is the fluid found between cells in tissues. It is blood plasma that does not contain plasma proteins, blood cells, and platelets. When tissue fluid drains into the lymphatic system, it is referred to as lymph. Here is how tissue fluid is formed. As blood enters the capillaries from arterioles, the smaller diameter results in a high hydrostatic pressure, so the plasma is forced out of the capillaries into the tissues where pressure is low. The blood cells, plasma proteins, and platelets are too large to pass through and so they remain in the capillaries, increasing the oncotic pressure inside the vessel. Towards the venule end of the capillaries, the oncotic pressure is higher than hydrostatic pressure. Some water is then reabsorbed into the capillaries by osmosis. The rest of the tissue fluid is absorbed into the lymphatic system and eventually drains back into the bloodstream near the heart.
The Heart
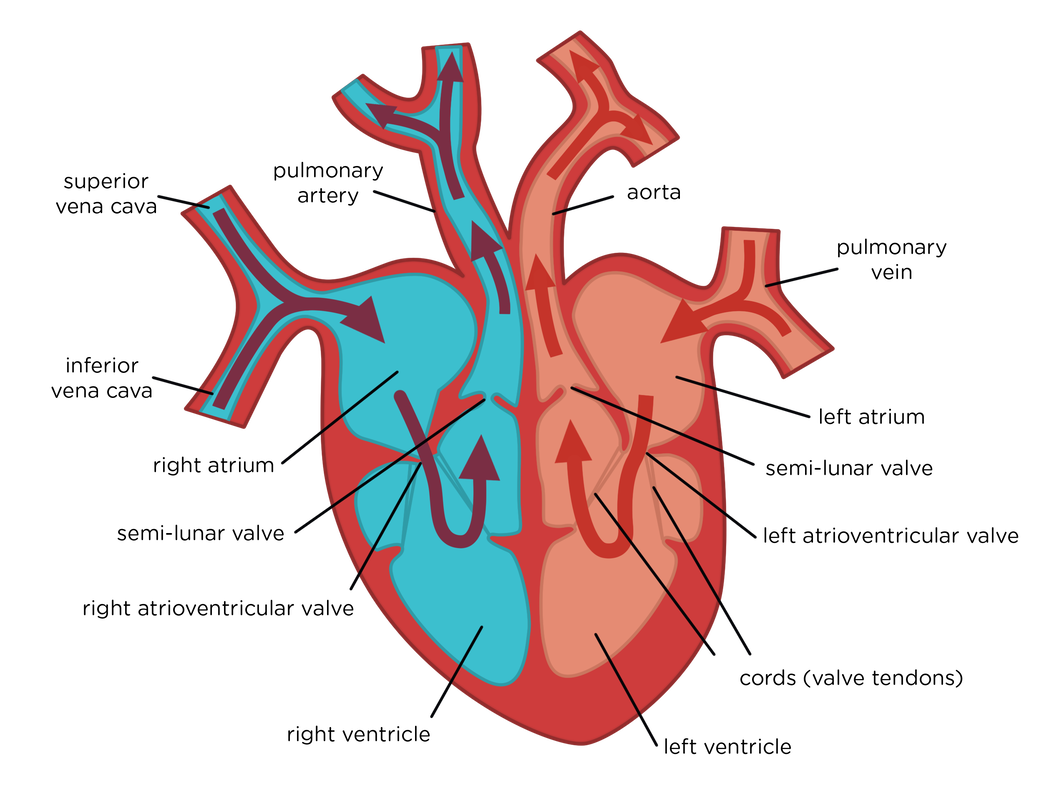
External Structure of the Human Heart
The human heart consists of two pumps that pump blood to different parts of the circulatory system. The pulmonary circuit re-oxygenate blood after returning from the tissues and is supplied by the right side pump. The systemic circuit delivers oxygenated blood to tissues and is supplied by the left side pump. The coronary arteries provide oxygen and nutrients to the heart tissue while the coronary veins remove the cellular wastes.
Internal Structure of the Human Heart
The right atrial and left atrial are thin-walled elastic chambers that receive blood from the veins and pumps it to the ventricles. In contrast, the ventricles are chambers with thick walls and pump blood out of the heart through the arteries. The left ventricle has the thickest wall to pump blood to the rest of the body at a higher pressure, whereas the right ventricle is much thinner as it pumps blood to the lungs, which is much closer to the heart. The atria and ventricles are separated by atrioventricular valves that stop the backflow of blood in the wrong direction. At the same time, the arteries leading from the heart are separated from the ventricles by semilunar valves that also prevent the backflow of blood.
Cardiac Muscle – The cardiac muscle can contract automatically without a signal from the brain. It is a specialized type of muscle found in the walls of the heart.
Cardiac Cycle
This is the series of events that happen within one full heartbeat.
Systole and Diastole
The heart contracts during systole and relaxes in diastole. When blood enters the right and left atria, pressure increases inside the atria. The atrioventricular valves open due to the higher pressure in the atria than the ventricles, and then the muscles in the atria contract pushing blood into the ventricles. This is known as atrial systole or ventricular diastole. Pressure increases inside the right and left ventricles when blood enters the ventricles. The atrioventricular valves close to prevent the backflow of blood into the atria. The pressure in the ventricles is also higher than in the aorta and pulmonary artery, forcing the semilunar valves to open. There is then the contraction of ventricular muscles and blood flows into the arteries. This is atrial diastole or ventricular systole. Atrial systole/ventricular diastole happens again. The pressure is higher in the aorta and pulmonary artery than in the ventricles. This leads to the semilunar valves closing to prevent blood from flowing back into the ventricles. Blood then flows into the atria, and the process repeats over and over again forming heartbeats.
The heart muscle can initiate its contraction and so it is said to be myogenic. The sinoatrial node (SAN) initiates a wave excitation in the left and right atrial muscles, causing contraction. The atrioventricular node (AVN) then conducts the wave with a slight delay to allow the atria to complete its contraction and passes it to the bundle of His. The bundle of His splits into the Purkynge tissue and causes contraction of the left & right ventricles from the bottom up pushing blood upwards towards the artery. (Transport in animals, 2016)
- Atrial fibrillation – atria beating faster than the ventricles
- Bradycardia – slow heart rate
- Ectopic heartbeat – irregular heartbeat
- Tachycardia – fast heart rate
Transport of Oxygen
Hemoglobin transports oxygen from the lungs to the tissues where it releases oxygen to be used. Hemoglobin binds four oxygen molecules. When hemoglobin binds to the first molecule of oxygen, it undergoes a change in shape increasing its affinity for oxygen. Thus, binding if the first oxygen increases the chances of the second oxygen binding, the third, and then the fourth. This is known as cooperative binding.
Carbon dioxide can also be transported in the blood. A little percentage of carbon dioxide dissolves in the blood; some bind to hemoglobin, forming carbaminohemoglobin while the highest percentage combine with water in blood-forming carbonic acid. When the concentration of carbon dioxide increases in the blood, it lowers hemoglobin’s affinity to oxygen. This phenomenon is termed Bohr Effect.
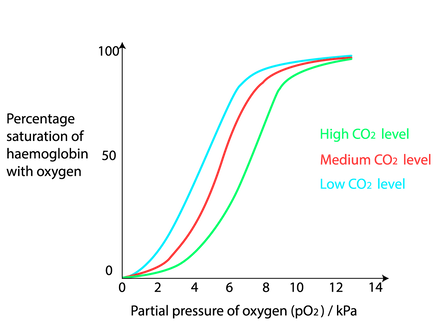
Cell Division
Cells divide by the process of meiosis or mitosis.
Cell Cycle
Different cells are at different points in their cell cycle. A lot of cells in multicellular tissue cannot divide anymore. The cell cycle describes a series of events that lead to the cell’s growth and replication of the genetic material to make identical daughter cells. These events can be grouped into three phases: interphase, mitotic (M) phase, and cytokinesis.
- Interphase – This is the phase in which the cells are not dividing and it is the longest stage in the cell cycle. Interphase can be split into three phases: G1, S (synthesis phase), and G2. The cell grows in size during the ‘G’ phases. There are checkpoints that ensure the cell is set to divide before it proceeds to mitosis.
- G1 – The cell is preparing to go to the S phase. These preparations include duplication of organelles, growth in size, and making proteins needed in the S phase.
- S phase – This is where DNA synthesis/ replication happens. At the end of the S phase, all the DNA has doubled in number and all the chromosomes are replicated.
- G2 – Here, the cell continues to grow in size, makes proteins, and stocks energy as it prepares for division. G2 ends when mitosis begins.
Cells that do not divide leave the cell cycle, for example, nerve cells. This occurs early in the G1 phase, where they can either proceed or go into the G0 phase. In the G0 phase, cells can differentiate into specialized cells, say muscle cells, die, or age.
Mitosis
This is where division begins and it results in the division of the nucleus. The chromosomes become more distinct and move to the opposite parts of the cell.
Cytokinesis
Cytokinesis is the process where the cell cytoplasm splits into two, creating two daughter cells. In animal cells, the cell membrane indents inwards until the two folds meet to separating it into two cells. This is different in plants where cellulose builds at the equator forming the endplate. The cell wall then forms at the endplate resulting in two plant cells.
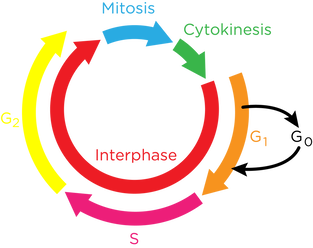
This process is repeated again and again, and so it is referred to as the cell cycle.
Checkpoints exist in the cell cycle to monitor the progress of dividing cells. They include:
- Restriction point or G1/S checkpoint- it checks if a cell has grown enough to pass from G1 to S phase
- G2/M checkpoint- before the cell enters the mitotic phase, this checkpoint checks and repairs DNA.
- Mitosis checkpoint- It is found in mitosis. It makes certain that a cell does not advance to the next mitotic stage before it is ready.
Interphase
In interphase, DNA is replicated. Each chromosome exists as a single chromatid, but it consists of two sister chromatids joined at the centromere after replication.
Mitosis entails four stages: prophase, metaphase, anaphase, and telophase. Mitosis involves the separation of sister chromatids to opposite ends of the cell so that the cell can divide.
Prophase
Before prophase, DNA is in long tangled threads, but it supercoils and shortens into a more dense structure during prophase. The nuclear envelope then disintegrates and breaks down. The centrioles divide and move towards the opposite poles of the cell. Centrioles play a function of forming spindle fibers, so spindle fibers form from the centrioles and make up the spindle apparatus. Plant Cells do not have centrioles, but they do have spindle apparatus that develop straight from the cytoplasm.
Metaphase
It involves the chromosomes lining up at the center of the cell. The chromatids become attached to spindle fibers at their centromere. They are then pulled along the spindle fibers to the equator of the cell and this prepares the chromatids for separation in anaphase.
Anaphase
The sister chromatids are split and, while still attached to the spindle fibers, are dragged to the poles of the cell with the help of motor proteins. However, this process requires ATP, so the mitochondria gather around the spindle fibers to provide it. When the chromatids arrive at the opposite ends of the cell, they are known as chromosomes. Anaphase results in each pole, having precisely the same number of duplicate chromosomes.
Telophase
Here, the spindle fibers begin to break down and the chromosomes begin to uncoil and lengthen; hence they become indistinct under the microscope. Then, the nuclear envelope reforms around the lengthening chromosomes. At the end of telophase, two nuclei are seen. The cell is now ready for the cytoplasm to split into cytokinesis to make two cells.
After telophase, the cell cytoplasm splits by cytokinesis leading to two new identical cells.
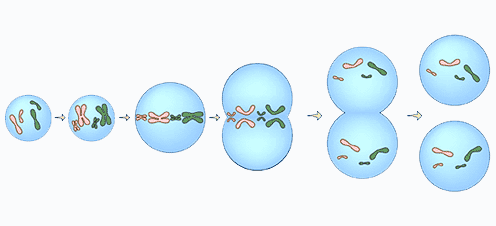
Meiosis
Meiosis is where four daughter cells are created with haploid nuclei-the gametes- and it does this through two divisions. The first division – meiosis 1 – comes just after chromosomes have duplicated in interphase. Here, homologous chromosomes are separated from each other. A short while after the first division, meiosis 2 happens. In meiosis 2, the chromatids in each chromosome are separated. At the end of meiosis 2, we end up with four genetically different cells, each with a haploid nucleus in them. This is due to variation created during crossing over and independent assortment.
Prophase 1
The chromosomes supercoil, the nuclear envelope disintegrates, and the spindle fibers appear. Then, the homologous chromosomes go through a process called crossing over. Crossing over is the exchange of DNA between chromatids on homologous chromosomes in prophase 1 of meiosis. The homologous chromosomes line up and twist around each other. The wrapping can cause sections of chromatid to break off; they can then be swapped over to the homologous chromosome. Because the chromosomes are homologous, crossed over chromosomes have the same genes as before. Genetic variation is introduced in crossing over since all of the alleles are shuffled around on different chromosomes.
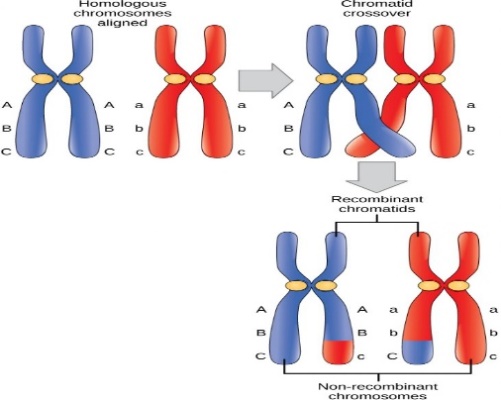
Metaphase 1
It involves chromosomes lining up at the equator. The first step involves the attachment of spindle fibers on chromosomes at the centromeres. The homologous chromosomes are then aligned at the equator. The homologous pairs are randomly arranged on either side of the equator, a phenomenon known as independent assortment.
Anaphase 1
The separation of homologous chromosomes to opposite poles happens. Each chromosome moves towards the opposite poles of the cell and consequently, each out of the pair of homologous chromosomes will wind up on opposite sides.
Telophase 1 and Cytokinesis
There is reforming of the nuclear envelope. Animal cells also undergo cytoplasm splitting in cytokinesis. Plant cells, on the other hand, go directly into prophase 2 without splitting.
Prophase 2
After meiosis 1, two sets of segregated remain. The chromosomes curl, the nuclear envelope breaks down, and new spindle fibers develop. The chromatids are no longer identical due to crossing over. This is what makes it different from meiosis.
Metaphase 2
Chromatids are randomly assorted onto either side of the equator through spindle fibers’ attachment on the centromeres.
Anaphase 2
The chromatids are pulled to opposite poles to the cell.
Telophase 2
The nuclei start to reform here and the cell splits in cytokinesis. In animals, the two cells split in two thus making four cells, while a plant cell divides four ways resulting in four daughter cells.
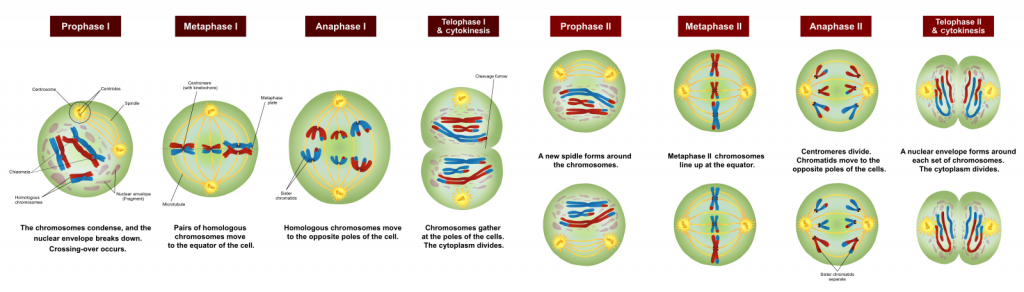
Stem Cells
Stem cells are cells that have not yet differentiated and can divide unlimited number of times by mitosis. When stem cells go through differentiation, they become specialized to perform specific functions. Fully differentiated cells are unable to undergo division, for example, nerve cells and muscle cells. Totipotent stem cells have the ability to differentiate into any type of cell. Stem cells that are pluripotent can form any type of cell apart from cells of the placenta. This are embryonic stem cells. Multipotent stem cells are adult stem cells that can differentiate into limited type of cells. Adult stem cells are important for growth and repair of the body and they may remain inactive for year; dividing and forming cells only when they are needed to. Unlike embryonic stem cells, adult stem cells are not suitable for use in research of stem cells. Stem cell therapy involves the introduction of adult stem cells to damaged tissue to treat disease. An example of this is the use of bone marrow transplant to treat leukemia.
Enzymes
Living organisms carry out a variety of metabolic reactions to survive. Metabolic reactions are all the reactions that happen in the body. These reactions need to happen really fast so that organisms can respond to changes in the environment. An increase in temperature can speed up these reactions, but very high temperatures tend to damage the cell in that; lipids melt under high temperatures. Because the cell membrane is made up of lipids, it will destroy the entire cell. Secondly, proteins are denatured at high temperature and this impends the functions of the cell.
Another way to speed up the reactions is to use a catalyst. A catalyst is a substance that speeds up the reaction rate but remains unchanged and reusable at the end of the reaction. Enzymes are an example of a catalyst and they work by lowering the amount of activation energy needed for a metabolic reaction to occur. Enzymes are proteins that catalyze chemical reactions that make life possible. Some of the essential reactions they catalyze are photosynthesis, respiration, and digestion. Enzymes are proteins but all proteins are not enzymes. A substrate is any molecule that can have its reaction catalyzed by an enzyme. The number of substrates an enzyme can catalyze in one minute is termed the enzyme’s turnover number. Substrates bind to the enzyme’s reactive part, named its active site, but the substrate only fits in the active site if it is the correct shape. So every single enzyme, and therefore every reaction has a specific enzyme to carry it out. An enzyme’s ability to achieve complementary binding with a substrate is due to the 3D shape of its active site.
n enzyme’s extracellular action occurs outside the cell producing the enzyme while intracellular enzyme action happens inside the cell producing the enzyme.
Activation Energy
For every specific reaction, there is an exact amount of kinetic energy needed to begin the reaction. This is called the activation energy. Lower activation energy leads to a faster reaction, whereas higher activation energy results in a slower reaction.
Enzymes reduce the activation energy needed for a specific reaction. This can be seen in the graph below.
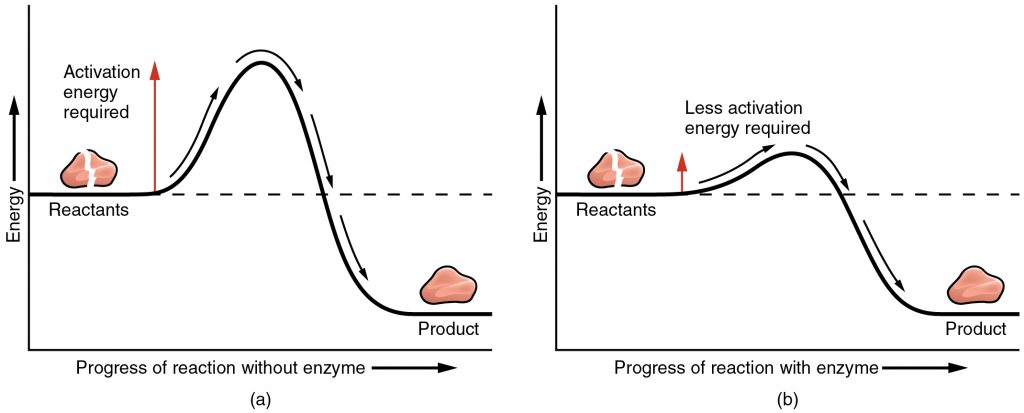
This action of an enzyme allows the metabolic reactions to happen faster without increasing the temperature and damaging the cells.
Active Sites
A substrate binds to the active site of an enzyme so that the enzyme can catalyze a reaction. On the active site, the substrate is held in a specific alignment that facilitates its reaction. The active site can also apply physical pressure to a substrate, leading to a reaction.
Properties of Enzymes
- Enzymes are neither consumed nor altered during a reaction.
- They are highly specific. Enzymes only bind to a specific substrate and catalyze a specific reaction.
- Several factors affect the speed of enzymes, such as temperature and pH.
Models of Enzyme Action
Lock and Key hypothesis
It proposes that the substrate is like a key that fits perfectly into the lock, the enzyme, owing to the complementarity in their shape hence the name lock and key. It says that the active site is in a never-changing shape and that the substrate can collide and attach to an enzyme forming an enzyme-substrate complex. A reaction then occurs and the products are formed. The products formed move out of the active site and the enzyme remains empty for another substrate to use.
Induced Fit hypothesis
It is a widely accepted model describing the way enzymes work. The enzyme active site is not exactly complementary to the substrate. In the presence of a specific substrate, a conformational change is induced on the active site making it complementary. When the substrate binds to the enzyme, the active site strains the bonds in the substrate, breaking them. This lowers the activation energy leading to a faster reaction. The products are then removed, and the enzyme active site can be reused by another substrate.
Factors Affecting Enzymes
- Temperature – Temperature increases the speed of the substrate and enzyme molecules by increasing their kinetic energy. This results in the substrate colliding with the active site more frequently, forming more enzyme-substrate complexes. The enzymes become inactive if the temperature is below zero degrees Celsius. If the temperature rises above the optimal temperature, the rate of reaction will slow down and eventually, the enzymes will be denatured. The rate of reaction also slows down when the temperature falls below optimal. For that reason, enzymes work best at an optimum temperature that ranges between 37 – 40 degrees Celsius.
- pH – Enzymes work best at a specific pH known as the optimal pH. All enzymes have their own optimum pH. Some work best in basic conditions, some in acidic while others in neutral conditions. An increase or decrease of pH from the optimum denatures the enzyme.
- Enzyme Concentration – An increase in the enzyme concentration leads to an increase in the reaction rate provided that the substrate is present in excess. That is, the reaction must be independent of the substrate concentration. The reaction rate increases owing to the rise in the number of available active sites.
- Substrate Concentration – If the amount of enzymes is kept constant, an increase in the substrate concentration leads to an increase in the reaction velocity until it reaches a maximum. Further increase in substrate concentration will result in the reaction velocity staying constant. This is because all the active sites of the enzyme are occupied.
- Enzyme Inhibitors – Enzyme inhibitors are molecules or substances that alter the catalytic activity of an enzyme by slowing down catalysis or stopping it completely.
Enzyme Inhibition
Enzymes can be deactivated by molecules called inhibitors. Inhibitors are any molecules that reduce or stop a reaction. Adding a small concentration of inhibitor will reduce the reaction rate, but if we add an inhibitor in excess, then the reaction may be stopped entirely. There are different types of inhibitors, like competitive inhibitors. A competitive inhibitor and a substrate have a similar physical structure, and so the inhibitor can bind to the active site. If it attaches to the active site, it will prevent the substrate from binding, thus preventing a reaction. This is because the substrate and the inhibitor are not identical and so the enzyme cannot convert it into a product. The enzyme and the inhibitor compete for the active site hence the name competitive inhibitors. Competitive inhibition can be overcome by increasing the substrate concentration. This is because, with more substrate than inhibitors, the substrates are more likely to collide with the active site forming enzyme-substrate complexes.
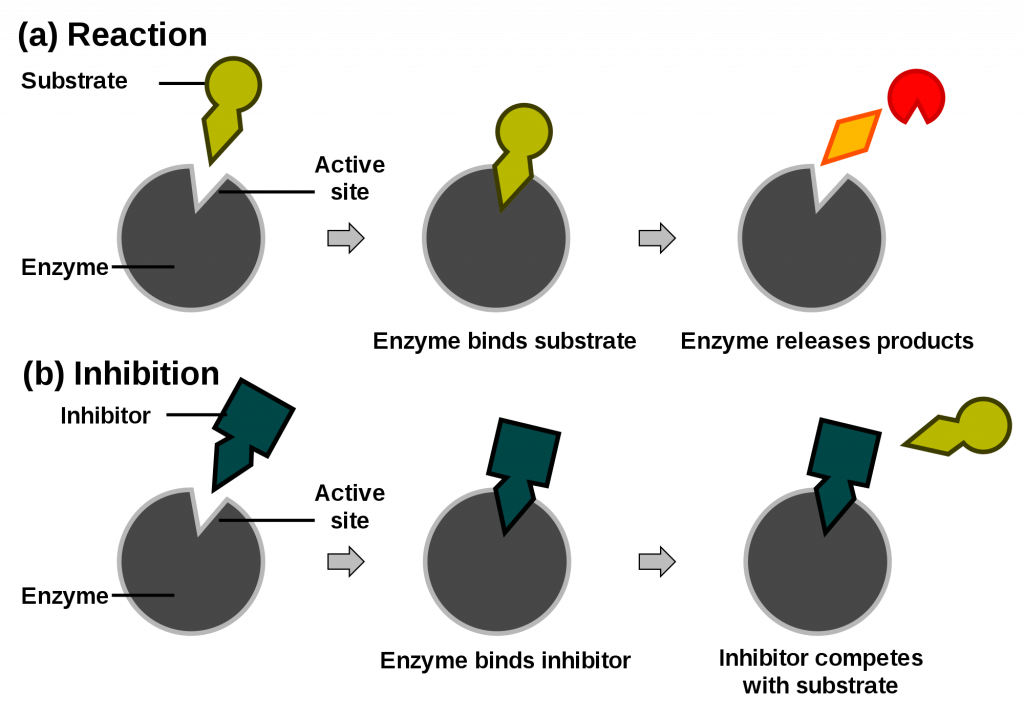
The other type of inhibitors is non-competitive inhibitors. The non-competitive inhibitor binds to a different part of the enzyme called the allosteric site, which is remote to the active site. The binding of the non-competitive inhibitor causes a conformational change in the enzyme. This alters the shape of the active site so that it is not complementary to its substrate and thus prevents the enzyme from converting the substrate into the product. Because these inhibitors do not bind to the active site, they do not compete with the substrate hence their name. Changing the substrate concentration will not affect this inhibition.
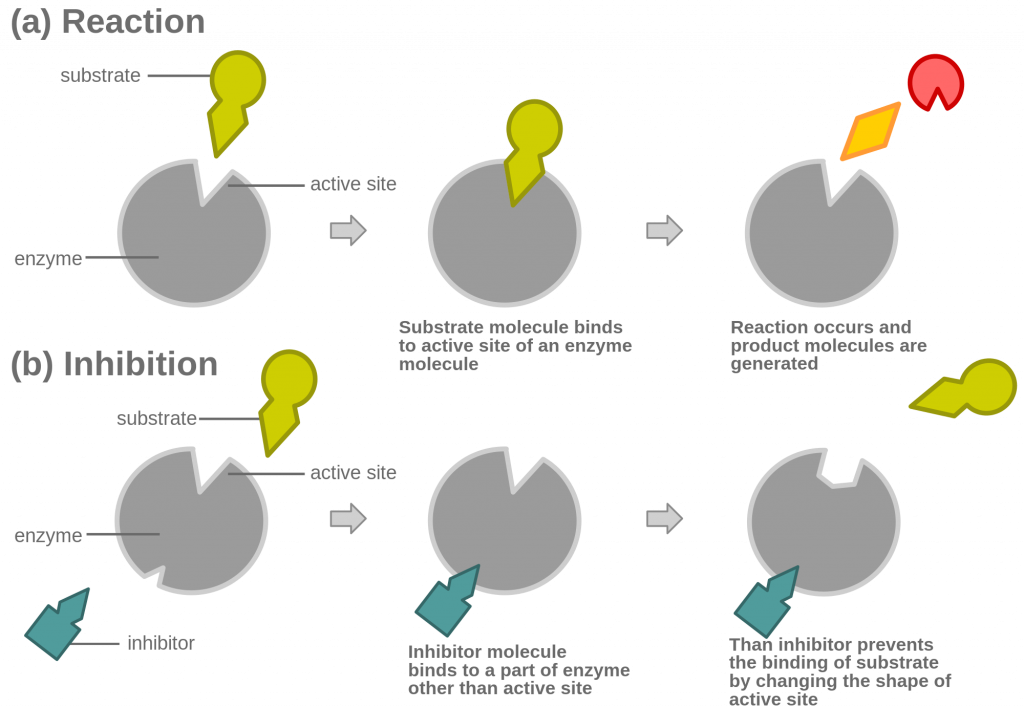
Comparing Enzyme Inhibitors
Competitive and non-competitive inhibitors work in different ways. The competitive inhibitor binds on the active site while the non-competitive inhibitor binds on an enzyme’s allosteric site. Competitive inhibitors bind reversibly to the enzymes, whereas non- competitive inhibitors bind permanently to the enzymes.
Reversible inhibitors bind to the enzyme using weak bonds, similar to those used in binding the substrate. These bonds are formed rapidly and break easily; therefore, the inhibition caused by these inhibitors can be overcome. Many drugs like anticancer drugs are reversible enzyme inhibitors. Irreversible inhibitors bind to the enzyme using strong covalent bonds than are hard to break. The inhibition created by these molecules is permanent. Irreversible inhibitors may include poisons such as sulphur.
Cofactors and Coenzymes
Cofactors and coenzymes are small molecules that are non-protein in nature and enhance the activity of an enzyme. Normally, they bind permanently or temporarily to an enzyme to form the active site. Coenzymes are organic molecules such as vitamins while cofactors are inorganic molecules, for example, metals like magnesium. Some enzymes cannot function without a cofactor or a coenzyme. When an enzyme combines with a cofactor or a coenzyme, it is called a holoenzyme.
Classification and Evolution
Classification can be defined as the process of placing living organisms into clusters. A scientist named Carl Linnaeus, about 250 years ago, published a hierarchical system that could be used to classify all species (Hammond, 2018)
The Modern Classification System
The organisms are classified into distinct categories with eight primary hierarchical levels. These are:
- Kingdom – the five kingdoms: Animalia, Plantae, Fungi and Protoctista, and the single-celled Prokaryota.
- Phylum – all organisms are organized based on their basic body design
- Class – the organisms are arranged based on their general characteristics.
- Order – this is where the organisms are sorted division based on more specific information.
- Family – this is a group of closely related genera.
- Genus – this is a group of closely related species.
- Species – it is the basic unit of classification in this system in which all members show little variation.
The reasons for classification of organisms are:
- For convenience
- To make the study of living things easier
- For easier identification of organisms
- To show the relationships between different species
Binomial Naming
In a global classification system, using a common name can be confusing owing to differences in language. The binomial naming system uses the genus name and species name to avoid confusion when naming species. So humans are Homo sapiens because they are in the species sapiens in the genus homo. Whenever a new species is named, it is given a scientific name based on its classification and a common name.
Reasons for the binomial naming system:
- The same organism may have a completely different common name in different parts of a country
- Translation of languages and dialects may give different names
- The same common name may be used for a different species in a different part of the world
- Classifying living organisms makes researching them much more manageable and helps in discovering new species.
Phylogeny
Phylogeny is the study of the evolutionary relationships among different groups of organisms. Individuals can also be classified using the phylogenetic system. This is a system that organizes species according to their evolutionary origins and relationships, and the more recent the ancestors, the more similarities there will be between the species.
N/B: Divergent evolution is where two species have evolved from a common ancestor, but the species get progressively less similar. Convergent evolution is where two species that do not share a common ancestor, who may share the same environment, evolve similar characteristics.
In a diagram representing the phylogenetic system, branches represent species while branching points represent common ancestors, and the closer the branches, the closer the evolutionary relationship.
A good classification system should be;
- Easy and clear to assign organisms into groups
- Containing a wide range of groups
- Using groups which can be categorized
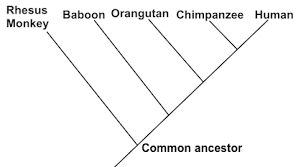
In this diagram, human, chimpanzee, orangutan, baboon, and rhesus monkey have a common ancenstor. A human and a baboon are more related than a human and a rhesus monkey. Nevertheless, a rhesus monkey is more related to a baboon than a human.
Evolution
Evolution is the change frequencies of alleles in a population over time.
Natural selection
Species have evolved to survive in the face of life-threatening conditions by the process of natural selection. Natural selection is an evolutionary mechanism that explains how specific features of the environment can apply a selective force on the reproduction of individuals within a population. It was Charles Darwin who proposed the mechanism of natural selection. Predation, competition, and diseases result in not all the members of a population surviving, thus the importance of natural selection.
Natural selection suggests that genetic mutations create multiple alleles of genes within a population, leading to genetic variation within the population. Genetic variation creates intraspecific competition. Individuals with particular genes can withstand difficulties imposed by the environment; therefore, these individuals with advantageous characteristics will be more likely to survive than those without the characteristics. Those that survive reproduce and pass on their favorable genes to the next generation; hence the next generation has a higher proportion of individuals with advantageous characteristics.
The most common evidence of evolution is fossil records. In the past, the world was inhabited by species that were different from those present today. Old species have died out and new species have risen, but the new species that have appeared are often similar to the older ones found in the same habitat. The similarity is confirmed by studying fossil records.
Example of natural selection
Giraffes have long necks that enable them to eat leaves from tall trees. It is believed that many years ago, giraffes had shorter necks and used to feed on shrubs. Over time the shrubs became scarce and some giraffes died due to lack of food. But some of them had undergone genetic mutation resulting in them having long necks and so these giraffes survived as they were able to feed on tall trees. They then reproduced and passed on their advantageous genes to the next generation. The ones with the shorter necks were eliminated, leading to a generation of giraffes with long necks.
Variations
Variation can be caused by a variety of genetic and environmental factors. Genetic variation can be caused by mutations, independent assortment and crossing over during meiosis, and the indiscriminate fertilization of gametes during sexual reproduction. Organisms from two different species show differences between them. This is known as interspecific variation. Members of the same species can also differ, and this is termed as intraspecific variation.
Focusing on a specific trait, there are two types of variations: continuous and discontinuous variation. Continuous variation is when there are two extremes and a full range of intermediate values in between, for example, height in humans. Characteristics that display continuous variation are often controlled by multiple genes and influenced by the environment. Discontinuous variation is where there are distinct categories with no intermediate values, for instance: gender in humans, which can either be male or female. It is not influenced by the environment and is regulated by a single gene.
Adaptations
Adaptation describes the characteristics which enhance the survival of an individual in a habitat. An adaptation can be anatomical, physiological, or behavioral.
- Anatomical adaptations – Anatomical adaptation deal with the structural features of an organism. For example, a fish has gills that enable it to breathe in water.
- Physiological/ biochemical adaptations – Physiological adaptations ensure the proper functioning of cellular processes. An example of this type of adaptation is seen in guard cells when they open when water is available and close as soon as the water is unavailable.
- Behavioral adaptations – This is the behavior of an organism that enables it to survive in its habitat. An example of this kind of adaptation is found in marram grass where when covered by sand, the marram grass will grow more quickly to reach the sunlight.
Changing Population Characteristics
Organisms keep on evolving as time passes. A species can be placed under new selection pressure and evolution will occur. This is more noticeable in the organisms with a shorter life cycle, such as insects than those with a longer life cycle like humans. An insecticide applies a very strong selection pressure and so if an insect is susceptible, then it will be wiped out, but if it has resistance to the insecticide, it will survive and reproduce, spreading the resistance through the entire population. For instance, Insect populations have become resistant to the insecticide DDT which binds to a receptor on the plasma membrane of specific cells in insects. This is due to mutations in the genes coding for cell surface receptors.
This is also seen in microorganisms. Antibiotics will kill most bacteria, but sometimes some become resistant. This may be due to the misuse of a certain antibiotic making the bacteria tolerant of the drug. As a result, they will multiply, bringing about evolution.
Immunity
Immunity is the ability of an organism’s body to recognize a pathogen, remove it, and destroy it quickly with effectiveness. A pathogen is a disease-causing organism. When pathogens infect an organism, it means they got past the natural defenses of the body (mucous linings, skin, and clotting scabs) and entered into the blood tissue. When antigens of body cells identify pathogens, they induce the production of antibodies. An antigen is a protein that produces an immune response by stimulating lymphocytes. The pathogen’s entry in the blood activates mast cells, which release two chemicals; histamine and cytokines. Histamine dilates the blood vessels making them more permeable. Dilation of blood vessels increases localized heat, which reduces the rate of reproduction of pathogens. Cytokines, on the other hand, attract phagocytes leading to phagocytosis. Phagocytosis is a non-specific immune response that engulfs and digests pathogens. A non-specific response means a response that is the same to all pathogens.
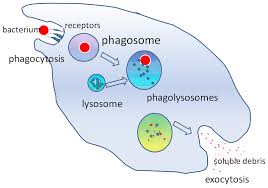
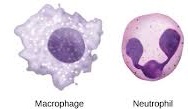
There are two types of phagocytes; neutrophil and macrophage. Neutrophil has a lobed nucleus, while macrophage has a round nucleus. The lobed nucleus enables them to squeeze out of blood vessels and attack pathogens on the infected tissue. Macrophages do not only digest the pathogen but also are antigens presenting cells. The macrophage detects the antigens on the surface of the pathogen, which are different from our cells. Small non -specific molecules called opsonins attach to various points of the antigens on the pathogen. The phagocyte then attaches itself to the pathogen, after which it engulfs the pathogen and encloses it in a large vacuole called a phagosome. The lysozymes enzyme from the lysosome of the macrophage breaks down the pathogen.
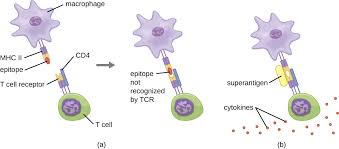
However, the pathogen’s antigen is saved during phagocytosis and moved to the surface of the cell membrane, so other immune cells can recognize the pathogen antigen. The non-specific response then interacts with the specific response. The interaction initiates a cell-mediated response. The response is carried out by lymphocytes (T lymphocytes). The phagocyte interacts with a T helper cell. The T cell has a specific antigen receptor called the CD4 receptor on their membrane, which binds with the antigen-presenting cell, stimulating interleukins production. Interleukins signal other T cells to multiply into; T memory cells responsible for immunological memory in secondary response, killer T cells that destroy antigen-presenting cells, and regulatory T cells that suppress the immune system.
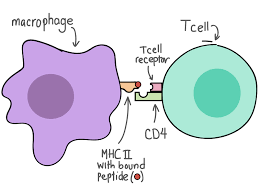
In addition, cells also stimulate the activation of B cells, which perform the humoral response. The humoral response is an immune response by the B lymphocyte cells. The B cells not only perform phagocytosis but also facilitate antigen presentation. B cell functions are determined by antibodies through a selection process called clonal selection. Their antibodies need to be complementary to the antigens. The humoral selection is divided into two parts; clonal selection and clonal expansion. Clonal expansion is the multiplication of B cells, which is later divided into B memory cells and B plasma cells. B memory cells form in the primary immune response when pathogens attack the body for the first time, antibodies are quickly destroyed but the memory B cells stay in the system so that during the secondary response, when the pathogen attacks for the second time, a lot more antibody is created in a short time. Besides, B plasma cells are responsible for making more cells with the same antibody to target the pathogens in the body.
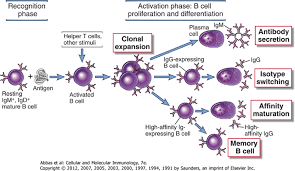
Antibodies are generally a family of proteins that specifically recognizes antigens. They are made up of heavy chains and light chains which are held together by disulfide bridges. The antibodies agglutinate pathogens together, forming a huge mass, making it easier for the macrophage and neutrophil cells to perform phagocytosis. They also act as antitoxins neutralizing toxins produce by pathogens.
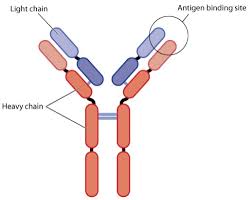
After all the pathogens have been destroyed, T killer cells destroy all the white blood cells on the site except T memory cells. Destruction prevents them from going into overdrive and killing other cells. Memory T cells are left alive for the future secondary response.
Transmission of Communicable Diseases
Communicable diseases can be transmitted either directly or indirectly.
Direct Transmission
This involves the transfer of microorganisms directly from an infected person to another. It can be through contact, inoculation, droplet transmission, or ingestion. Contact transmission can be through touch or when blood or body fluid from an infected person comes into contact with the mucous membranes of an infected person. Pathogens can also enter the body of a host through a break in the skin of the host. This is known as inoculation. Ingestion of contaminated food can also result in the transmission of microorganisms from the hand to the mouth. Droplet transmission involves the transfer of small droplets of saliva released when an infected person coughs, sneezes, or coughs to another person’s mucous membrane. It requires people to be in close proximity to each other. Tuberculosis and influenza are some of the diseases transferred by droplets.
Indirect Transmission
This involves the transfer of microorganisms from an infected person to a person who is not infected by means of a fomite or a vector. A fomite is an inanimate intermediary that transfers pathogens from an infected individual to a susceptible individual, such as pencils, clothing, or doorknobs. At the same time, a vector is an animate intermediary that carries pathogens to a susceptible person such as mosquitoes.
Portals of entry of pathogens into the body include the digestive tract, mucous membrane, respiratory tract, genital tract, or the skin.
Prevention and Treating communicable diseases
There are many ways in which the spread of infectious diseases can be controlled. One of the most common ways is modifying the environment. This includes improving sanitation and hygiene in our surroundings, ensuring food safety, vector control such as draining stagnant water to prevent the breeding of mosquitoes, improving housing and working conditions, and ensuring water safety, leading to a reduction of waterborne diseases such as cholera. Another way of preventing the transfer of communicable diseases is by changing behavior. This can be regular handwashing to reduce food contamination, using condoms to prevent sexually transmitted infections, using insecticides to kill mosquitos, or washing fruits and vegetables before consuming them.
Vaccines have led to a decline and, in some cases, eradication of communicable diseases like smallpox. Immunization in children has resulted in a reduction in diseases like whooping cough, mumps, and polio. When almost all the members of a population are vaccinated, precisely above 80%, other unvaccinated members of the community are also protected. This is known as herd immunity. Surveillance is also used to control infectious diseases. It is used to monitor diseases, characterize disease patterns, and detect outbreaks. It can also guide the evaluation and prioritization of disease control programs. Health facilities require effective ways of controlling communicable diseases as they can spread rather quickly in these facilities. Ways to prevent the spread of infections in these amenities include: wearing personal protective equipment such as gloves and masks, washing hands, proper sterilization of apparatus, maintaining environmental cleanliness, and isolation of infected patients.
Medications have been one of the main ways of treating and managing infectious diseases. Antibiotics work by killing microorganisms or stopping them from dividing. The current problem in treating infectious diseases is antibiotic resistance. Antibiotic resistance has become widespread due to the misuse of these drugs. The microorganisms have developed tolerance to the drugs, thus the resistance.
Plant Defences
Passive defenses
Plants have a thick and impenetrable bark that prevent the entry of pathogens. They also have a waxy cuticle that prevents water from collecting on leaves, thus hindering the survival of microbes. Plants have a cellulose cell wall that also plays the role of a barrier. They also contain lignin in the cell wall which is technically indigestible. Plants also have cellulose blocking the sieve tubes to prevent the spread of microorganisms throughout the plant.
Active defenses
- Plants close their stoma to prevent the entry of microorganisms.
- They also deposit cellulose at the cell walls strengthening the cell walls
- They increase the number of oxidative oxygen bursts to kill pathogens when they enter the cell.
- Plants can also induce cell necrosis.
- They also produce various chemicals with antimicrobial properties that kill pathogens.
References
Hammond, A. (2018). Classification and Evolution. Weebly. Web.
Imperiya.by. (2019). The Mammalian Heart. Web.
Khan Academy. (2015). Cell Theory. Structure of a cell. Biology. Khan Academy. Web.
Transport in Animals. (2016). A-levelsnotes. Web.