The Rationale
Industrial-scale desalination plants cannot be scaled down for use in remote areas due to the complexity of the technology. Therefore, the need for scaled-down desalination plants to meet specific consumer needs for clean water particularly in remote desert and semi-desert areas cannot be overemphasized. That reinforces the need to research tested and approved technologies. That is further reinforced by the fact that different technological innovations that use solar energy to desalinate seawater have emerged in the recent past (Manwell & McGowan, 1994). Desalination technologies are applicable in different environments with different background solar radiation. These solar-powered thermal desalination plants rely on the technique of thermal collectors as the source of heat. The solar collector mechanism is briefly discussed below followed by a detailed discussion of new technological advances (Manwell & McGowan, 1994).
Characteristics of Solar-powered Thermal Distillation Machinery
The nomenclature of solar-powered thermal distillation machinery is characterized by a heat absorber that is made of either aluminum or copper. Comparatively, aluminum has overtaken copper since it has a higher thermal conductivity than copper. Other characteristics include radiosity which is determined by the region where the desalination plant is located, the thermal conductivity of the conducting materials, the cross-sectional area of the thermal conductors which determines the amount of exposure to the sun, and the thermal energy absorbed due to that exposure. The heat capacity of the input fluid and heat effects are other characterizing variables.
Technology
One of the technologies that have been developed which use the principles of thermal desalination is the solar thermally driven stand-alone desalination system. The system has an estimated capacity of 20 m³/d and uses a membrane distillation (MD) technique. The system’s integral components are internal and external heat recovery mechanisms that use high-efficiency thermal collectors as the source of heat. The thermal collector is resistant to the corrosive effects of seawater, a critical advantage of the system. A typical economic evaluation of the system indicates that expensive components such as the heat exchanger are not incorporated into the design (Ophir & Lokie, 2005). Besides that, no pump is used in the design, guaranteeing a further reduction in the use of costly components. On the other hand, cost savings due to the control unit used by the collector loop is technically feasible (Ophir & Lokie, 2005).
The MD Technique
The membrane distillation (MD) technique is based on the principles of partial pressure. Typically, the system consists of membranes with a pore diameter of 0.2 µm made of a hydrophobic polymeric material that separates the membranes. The separation is designed to enable the system to achieve the partial pressure required to wet the membranes. However, the system reaches a limiting pressure beyond which wetting does not occur. Water molecules pass through the membranes in the form of steam as illustrated below.
Condenser foil
A description of the principles of operation of the membrane can be analyzed using a typical component of a desalination machine. In the setting, seawater at a temperature of 80 ºC is used on one side of the membrane while on the other side of the membrane the temperature is at 70 ºC. The temperature difference between both sides of the membrane causes partial pressure to be experienced causing water to evaporate through the membranes. Finally, a low-temperature distillate is formed at the low-temperature side of the membrane (Qiblawey & Banat, 2007).
A critical analysis of the application of the system calls for an evaluation of the environment in which the system is intended to be used. In practice, semi-desert and desert environments do not get any power supply from the grid systems, hence relying on the solar energy that is available through radiation. The system has to be cost-effective and be energy efficient to be applied in desert or semi-desert areas. That calls for the need to incorporate the concept of an efficient heat recovery system to drastically reduce the demand for extra energy is critical. Therefore, a heat recovery system must be integrated by the use of either an internal or external heat exchange mechanism with the feed water acting as a coolant for the system.
An examination of the principle of the internal heat recovery mechanism is illustrated in the diagram below.
The rationale of the internal heat recovery mechanism is to reinforce the need to efficiently prevent and recover any heat losses in different climatic conditions. However, the system can be used in semi-desert and desert conditions with special emphasis on remotely inaccessible areas where the supply of electricity from the national grid system is not possible (U.S. Department of the interior, 2002).
The technology integrates the use of three channels consisting of interchangeably organized condensers at different intervals with the evaporator and the distillate. An impermeable compartment consisting of the condenser foil separates the condenser and the distillate in the system. Typically, the functionality of the system is discussed here. Assume that the hot water used in the above demonstration is also used in the current setting. The hot saline water moves along the system membrane passing through the condenser channel, causing an aggregate warming effect, and eventually dropping the temperature to 75 ºC from 80 ºC. The temperature difference between both sides causes some partial pressure to be experienced causing steam to flow throughout the membrane (U.S. Department of the Interior, 2002).
Typically, the condensation of water along the foil causes the heat from the evaporation to be transferred to the flowing water, thus recovering the heat in the process. It is important to note that the temperature of brine due to the saline water drops as a result of the recovery of heat from the system. A critical analysis of the system indicates that the liquid distillate is obtained at the outlet of the system where the difference between the inlet and outlet temperature is 5º C. That is meant to create the desired temperature gradient (Office of Saline Water, 1971).
A critical analysis of the thermal efficiency of the system indicates that the volumetric flow rate coupled with the temperature gradient of the system defines the thermal efficiency of the system. Typically, the thermal efficiency of the system depends on the output ratio (GOR) gained by the system and is calculated based on the latent heat of the system and the input energy (Mcelroy, 1993).
To evaluate the satisfaction derived from the technical and operational feasibility and efficiency of the system, it is important to note that the system has been tested on a small scale with satisfactory results. The system that was tested consisted of an MD component, a solar collector designed to be corrosion-resistant, a pump and a hysteresis controller, and a number of sensors for detecting temperature, volume flow, and pressure changes as experimental variables (Lu, Walton, & Hein, 2002). When the system performance was analyzed, it was established from experimental investigations that the system was flexible to handle and had the advantage of low maintenance cost coupled with high operational efficiency (Mcelroy, 1993).
A critical evaluation of the system during the investigation indicated that the operating temperature of the system was compatible with the optimum operating temperature of the solar collector. The optimal operating temperature of a solar collector lies between 60 ºC and 80 ºC, a critical advantage of the system.
In a typical operating environment, fouling and scaling effects are bound to occur which could adversely be affecting the overall performance of the system (Al-Shammiri & Safar, 1999,). However, the system has been tested and found to be free from fouling and scaling effects. On the other hand, chemical feed pre-treatment is not required as integral components of the system. Another technical and functional benefit of the system is that the membranes are never in danger of getting damaged due to crumbling when they become dry. On the other hand, high-quality water and system efficiency are additional factors that reinforce the cost-benefit analysis of the system’s economic and technical feasibility.
The need for a critical study of other desalination systems that have been developed most recently is vital. That is typical because of the call for a reduction in capital investments and the cost of desalinated water targeting users in semi-desert and desert areas. One of these technologies is the Low-Temperature Multi-Effect Distillation (LT-MED) (Barron, 1992).
Low-Temperature Multi-Effect Distillation (LT-MED)
The efficiency of the Low-Temperature Multi-Effect Distillation (LT-MED) has been tested, evaluated, and economically proven to be efficient, calling for a critical discussion of the system as a viable option. An important characterizing element is its good thermodynamic functionality. That is coupled with significantly low-pressure drops accompanied with high volumetric efficiency of vapor flows providing economic feasibility of using low-cost durable materials (Barron, 1992).
A critical evaluation of the systems indicates that the technical construction of the system using aluminum alloys that provide an efficient transfer of heat within the system ups its operational efficiency. That is typically due to the fact that the thermal conductivity of aluminum is higher than that of copper, making the material specifically suitable for providing superior thermal functionalities (Office of Saline Water, 1971)
In the construction of a typical system, aluminum provides a higher heat transfer per ton of water than copper when the same investment cost is calculated for the two materials. That has the overall effect of causing an effect on temperature drop to be lower when using aluminum, allowing for the incorporation of a bigger number of effects for an equivalent investment of aluminum compared with copper. In practice, the economy ratio is very high as has been proven with similar plants that have been used at the Virgin Island plants (Barron, 1992).
Another merit reinforcing the use of aluminum over copper is to make optimal use of the energy component where low-grade and low-cost heat can be used efficiently by making it available through cogeneration schemes. The technology incorporates inexpensive centrifugal compressors which demand minimum inputs for pre-treatment machines (Barron, 1992).
Consider a Multi-Effect Desalination (M.E.D.) plant. Established MED plants are constructed using horizontally arranged aluminum alloy tubes with an evaporative condenser that consists of falling films in a serial arrangement (Al-Shammiri & Safar, 1999).
The arrangement of tubes defines the operational efficiency of the system. The tubes are serially arranged to optimize the production of multiple amounts of distillate in a repetitive process involving evaporation and condensation. Several evaporative condensers are used for the recovery of heat in the process. However, the system can be optimized with the latter arrangement. The availability of low-grade heat and the investment cost is influencing variables that determine the optimal efficiency of the system. Technically, the temperature difference between the steam and the inlet temperature of seawater affects the overall number of effects. The minimum temperature difference allowed for each effect is also influenced by the arrangement of the aluminum tubes (Barron, 1992).
Operationally, the input seawater is divided into two streams at the inlet after it has been deaerated and preheated. At this point, one stream is allowed to flow into the sea as a coolant, and the other acts as the input source for desalination. The heating process is done at the heat rejection condenser. Afterward, the water to be distilled is allowed into the lowest temperature compartment, referred to as backward feed flow. That is influenced by the need to optimize the thermodynamic efficiency of the system which is achieved by controlling the mixing of colder seawater with the higher temperature effects (Barron, 1992).
Other technologies
It is important to note that the source of power for thermal energy is solar energy. However, it is important to discuss further other solar thermal desalination technologies to crystallize the most economically feasible technology that can be widely applied in semi-desert and desert conditions with technically proven operational efficiencies. The technology employs direct solar desalination techniques that have been modified. Typical systems include basins still, diffusion, and wick stills, among others. Basin still is characterized by single slopes, cover cooling, and a condenser. On the other hand, a wick still is made of radiation absorbing elements to absorb thermal energy for use in heating the saline water. These technologies use harnessed solar energy to provide thermal energy. The solar energy sources include a parabolic trough collector, evacuated tube collector, and Salinity-gradient solar ponds, among other solar technologies (Mallikarjunaiah, 1988).
Salinity-gradient solar is designed with a shallow pond where desalinated water remains at the top of the salty water while the salty water settles at the bottom of the bond, a process caused by a vertical differential gradient in the concentration of salt. The technique causes water at the lower end of the pond to attain a temperature range of 70 ºC and 80 ºC (Mallikarjunaiah, 1988).
Another solar technology that can be integrated into the system is a flat plate collector which is a technique used to transfer heat using heat absorption pipes. The system assembly consists of transparent pipes that are arranged on a flat surface which are coated to minimize heat losses and optimize the absorption of incident radiation (Mcelroy, 1993).
Another technology that has been operationally tested and found to be effective is the evacuated tube collector. Here, heat losses are minimized by an evacuated receiver. The technology is either designed with two coaxial tubes with seals at both sides and glass to a metal seal with a metallic receiver. Comparatively, evacuated tubes provide better operational efficiency compared to flat plate collectors. Evacuated tubes are more expensive than flat-tube collectors. Despite that disadvantage, evacuated tubes require less surface area usage than flat tubes. One of the solar collector technologies is the parabolic trough collector. Its principle of operation is discussed below (Mcelroy, 1993).
The Parabolic trough collector
The technology has a linear collector consisting of a parabolic cross-section. Solar energy in the form of light is concentrated by the solar reflectors into the focal point of a receiver tube where the fluid in the tube is heated and transferred to the designated point for desalinating input water. It has been demonstrated through theory and practice that the parabolic trough collector is more economically feasible for desalination purposes compared with other techniques (Mireles, 1991). In addition to that, solar ponds are widely used technology for desalination, though the parabolic trough collector provides a higher thermal efficiency compared with solar ponds technology (Mallikarjunaiah, 1988).
Comparatively, solar collectors are very expensive and it is recommended that solar ponds be the most suitable method for use where land is in plenty and cheaper (Mallikarjunaiah, 1988).
Another solar desalination technology that has received continued improvements is direct solar desalination. However, this technology has evolved through widespread modifications. The modification consists of a solar collector attached to a hot water storage tank with a desalination unit. This configuration relies on natural confections to achieve the distillation objective. The technology of the parabolic trough collector’s principle of operation is discussed below.
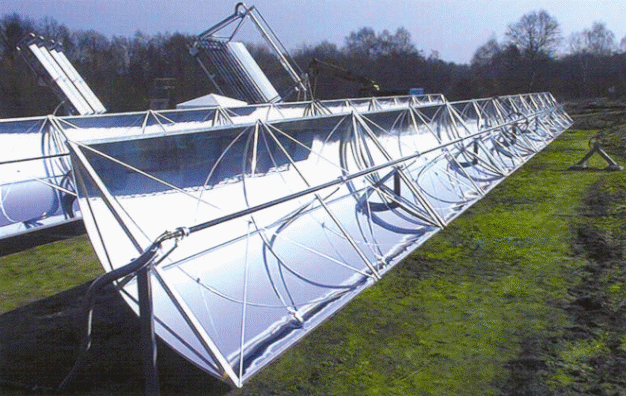
Picture of a practically installed optical collector.
Principle of operation
The performance of the above system has been tested for its appropriateness in tapping solar energy. Findings were represented graphically as shown. Moreover, the experiment was done at TRY of Copenhagen.
The technology was satisfactorily proven to work even in colder climates such as in central Europe. Technically, the collector was identified to be characterized by economic and energy efficiency. However, performance improvements of the technology were based on low-cost high reflector collectors, minimal thermal losses due to the use of specialized coatings, and other minimum design improvements (Mcelroy, 1993).
However, the system calls for further improvements to optimize its efficiency in the collection of solar power. The improvement should target enhancing the reflectivity of optical reflectors, incorporating end-loss optical reflectors, and a thorough investigation of the performance of the optical systems.
The Economic Rationale
The economic efficiency of solar-powered thermal desalination plants revolves around the fact that investment capital on water costs is reduced by 8% and 17% for desalination water, facts established from research that has been conducted on the technical and economic feasibility of solar-powered thermal desalination plants (Mireles, 1991). Further economic facts indicate that MED has the advantage of offering further reductions in costs, a comparatively more efficient technology compared with the others discussed above (Swift, 1988). To further realize the benefits and optimize MED and other newly evaluated systems including LED-MED, researchers should factor the use of improved antiscalants and multiple wetting techniques factoring in the use of intermediate pumps that rely on solar power to function (Glueckstern, 1995).
The internal rate of return (IRR) on such an investment in the costs-benefit analysis mechanism has been demonstrated from research findings to be 8.5% less costly compared with the use of gas as an economic favorability particularly in cold countries ( Mireles, 1991). However, it is important to conduct an environmental impact assessment of the adverse effects of desalination when selecting the most appropriate technology to use (Morton, Callister & Wade, 1996).
References
Al-Shammiri, M. & Safar, M., 1999, Multi-Effect Distillation Plants: State of the Art, Desalination, 126 (1999): 45-59. Web.
Barron, J. M., 1992, Installation and Operation of A Multi-Effect Multi-Stage Separator Coupled with A Solar Pond, Master’s Thesis from University of Texas at El Paso Mechanical Engineering Department.
Glueckstern, P., 1995, Potential Uses of Solar Energy for Seawater Desalination, Desalination, 101 (1995): 11-20.
Lu, H., Walton, J. C., & Hein, H. 2002. Thermal Desalination using MEMS and Salinity-Gradient Solar Pond Technology. Desalination Research and Development Program Report No. 80. Web.
Mallikarjunaiah, K.J. 1988, Performance of a Solar Pond Coupled Multistage Flash Desalination System, Master’s Thesis from University of Texas at El Paso Mechanical Engineering Department.
Manwell, J. F. & McGowan, J. G., 1994, Recent Renewable Energy Driven Desalination System Research and Development in North America, Desalination, 94 (1994): 229-241.
Mcelroy, R. I., 1993. Mechanical and Thermodynamic Performance Analysis of a Multistage Desalination System, Master’s Thesis from University of Texas at El Paso Mechanical Engineering Department.
Mesa, A. A., Gomez, C. M., & Azpitarte, R. U. 1996, Energy Saving and Desalination of Water, Desalination, 108 (1996):43-50.
Mireles, E.P. 1991. Economic Feasibility of Utilizing Solar Pond Technology to Produce Industrial Process Heat, Base Load Electricity, and Desalted Brackish Water, Master’s Thesis from University of Texas at El Paso Mechanical Engineering Department.
Morton, A.J., Callister, I.K., & Wade, N.M. 1996, Environmental Impacts of Seawater Distillation and Reverse Osmosis Processes, Desalination, 108 pp. 1-10.
Ophir, A. & Lokie, F. 2005. Advanced MED Process for most economical seawater Desalination. Desalination, (182), pp. 187-198.
Qiblawey, H.M., Banat, F. 2007. Solar thermal desalination technologies. Web.
U.S. Department of the Interior. 2002. Thermal Desalination using MEMS and Salinity-Gradient Solar Pond Technology. Web.
Office of Saline Water, 1971, Saline Water Conversion Engineering Data Book, Second Edition, United States Department of the Interior. Swift, A.H.P., 1988, Solar Engineering 1988, ASME (1988): 14-16.