Hypothesis
Life cycle cost drivers do not influence the whole life cycle in engineering asset management.
Literature Review
To collect academic sources (articles published in academic journals) about life cycle cost drivers and engineering asset management, a literature review was carried out. As a result, it has been investigated which issues have already been discussed and reflected in the existing body of literature and which problems remain unsolved. After the search had been made, the most relevant articles were selected.
Life Cycle Costing
Having appeared in the 1960s, life cycling costing has changed significantly from military aims to more general system design considerations, i.e. to assess and compare relative benefits of different options (Love et al. 2013). Throughout the long process of using life cycle costing in a wide range of spheres, there is no agreement in terms of the exact definition. Following Blanchard and Fabrycky (2011), it is possible to understand the life-cycle as the period spanning from the phase of acquisition through the utilization phase, starting with conceptual designs and finishing with product disposal. This conception is reflected in present-day research.
In the process of life cycle development phases, operating and economic issues are taken into account using contrasting the cost of the product design with a certain reliability level (warranted period, useful product life, expected product life) and the support costs for some time after this product is received by the customer (El-Akruti & Dwight 2013). It is argued that life-cycle costing and engineering are not similar to other costs and procedures since the whole lifecycle is considered in each phase of the system development when using life-cycle costing (He & Xiong 2013). Because the primary attention is drawn to long-term consequences, saving money, and buying low-cost assets is not considered an adequate strategy in the overwhelming majority of cases (Love et al. 2013).
Life Cycling Costing and the Design Phase
While most costs of a final product are materialized in further stages (manufacturing and distribution), it is generally agreed that these costs are nevertheless determined in the earlier phases (Vikhorev, Greenough, & Brown 2013). Consequently, the most effective way to control costs is to identify cost-related issues in the design phase (Zakeri & Syri 2015). In this respect, the task of primary significance for designers is to understand the connection between the known cost information and decisions. Having realized the importance of early cost commitments, managers and engineers should examine the decision-making process throughout the design phase and take into account the way particular decisions can influence total life-cycle costs (Ristimäki et al. 2013). Ristimäki et al. (2013) state it is likely to become a challenge since there is an inverse relationship between the accuracy of the cost estimates and the period between the estimate and the actual event. The reason for this is quite simple: due to the lack of information including the uncertainty about the system plan, it is difficult to assess a potential situation.
In designing and predicting costs for the life-cycle of a system, the projected life of goods is considered: it includes product and market research, design phases, manufacturing, product reliability, and maintenance and support issues (Zakeri & Syri 2015). There are diverse approaches to the costs that should be taken into consideration in the life cycle. For instance, some researchers suggest the idea that marketing and disposal costs must be considered although other studies do not review them (Blanchard & Fabrycky 2011). As a result, it is necessary to specify the phases and costs included in the life-cycle cost forecasts in each particular case.
Tradeoff Research in Life Cycle Costing
One of the most important components to guarantee the implementation of an advantageous system configuration is trade research that is concerned with quantifying various options. The complete life-cycle of the product is controlled in all phases of the product development, and technical considerations, as well as the economic ones, should be viewed to provide comparing design costs and maintainability (Hasan, Hossain, & Habib 2015).
In modern academic literature, the concept of the trade space as the set of system parameters, attributes, and characteristics needed to meet the performance requirements becomes important (He & Xiong 2013). Through this perspective, it is stated that the decision-makers theoretically define and refine the developing system and make tradeoffs taking into account cost, schedule, risk, and performance (He & Xiong 2013). Although this issue is now acknowledged as one of the most relevant and organizations tend to weight the importance of different criteria, the numerous articles fail to give sufficient examples that can demonstrate whether trade studies may be successfully practiced in life-cycle costing (Hasan, Hossain, & Habib 2015).
Cost Modelling
Some authors point out that, apart from evaluating different system options, it is essential to explain costs in life-cycle costing (Karniouchina et al. 2013). Systems costs should be decreased so that the management is provided with the visibility demanded in estimating different facets of system design and development, production, operational use, and support (Karniouchina et al. 2013).
There are several cost models, simple and complex; it is probable to distinguish conceptual, analytical, and heuristic ones. Conceptual models are composed of a set of hypothesized relationships expressed in a qualitative framework; as a rule, they are flexible and suit various systems (You et al. 2012). In comparison, analytical models normally relate to mathematical relationships designed to examine a certain aspect of a system under special conditions (You et al. 2012). Finally, Heuristic models are less structured analytical models; an approach providing a plausible solution is used, but the models may apply only to concrete cases for which they have been created (You et al. 2012). Thus, every model has its limitations, and it is necessary to consider the situation before choosing one of them.
Overall, the literature review demonstrates that life cycling costing affects systems costs, and early phases are significant. However, it is not clear how life cycling costing is viewed by different specialists.
Annotated Bibliography
Yue, D, You, F, & Darling, SB, 2014 ‘Domestic and overseas manufacturing scenarios of silicon-based photovoltaics: life cycle energy and environmental comparative analysis’, Solar Energy, vol. 105, no. 3, pp. 669-678.
In the present article, the authors examine the life cycle assessment as one of the most effective instruments to estimate energy and environmental profiles of a photovoltaic (PV) system. They review these issues about the protection of the environment and explain the necessity to find more sustainable technologies. As they have been commercialized and popularized, silicon-based PV technologies become the subject of the researchers’ interest. It is pointed out that the large body of life cycle assessment literature focuses on Europe and North America while other countries, such as China, have not been properly studied in this context. The importance and relevance of PV systems are proven via the literature review. The rapid changes in these spheres are emphasized as one of the most significant reasons for conducting studies in this area.
The authors aim to carry out a comparative life cycle assessment using domestic and foreign manufacturing scenarios exemplified by three types of silicon-based PV technologies: monocrystalline silicon, multi-crystalline silicon, and ribbon silicon. In this respect, the life cycle methodology is underlined, and foreign manufacturing scenarios are identified. It is peculiar that the main kinds of life cycling assessment measures are addressed: process-based methods, input-output (I/O) analysis, and hybrid life cycle assessment methods are described and explained. Besides, the authors define the key terms, such as the functional unit, that will be necessary for this study. Later on, the researchers continue with the life cycle limitations and inventory; energy and environmental profiles are discussed on the ground of particular indexes.
The suggested life cycle energy profile consists of two sections shedding light on the constituents of this particular life cycle system, energy payback time, and energy return on energy investment. Further, the carbon footprint outcomes for different silicon-based PV technologies are given.
The authors of the article consider geographic diversity by using local inventory data for a wide range of processes and resources. In the course of their research, they thoroughly analyze the energy payback time, energy return on investment, and greenhouse gas emissions. By the results provided in this article, the energy usage efficiency is de facto 30% lower in contrast to the national manufacturing development, and the carbon footprint is about twice as much in the foreign manufacturing. These results demonstrate that a break-even carbon tariff model intends to make use of the universal silicon-based PV modules.
The article is well-thought and structured. The logical division of ideas and illustrations add up to the quality of the paper and comprehension. Moreover, some issues correspond to the textbook by Blanchard. The concept of “system life cycle engineering” becomes one of the most significant parts of the performance (Blanchard, BC & Fabrycky 2011). It is emphasized that one would be unable to understand the mechanism of life cycles and the role of cost drivers without it.
Asset Life Cycle Aspect
In their article, Yue, You, and Darling (2014) address a wide range of aspects connected with life cycle costs and PV systems producing electricity directly from solar radiation. As a system is composed of human resources, products, and procedures that allow satisfying the identified needs, all these constitutes are addressed. The authors’ approach correlates with the idea expressed by Blanchard and Fabrycky (2011): it is important to make life-cycle considerations a specific aspect of the feasibility analysis process. This section of the paper examines one of the products discussed by the authors, Si-PV modules, that are to become the basis of the new approach towards energy usage and consumption.
First and foremost, Yue, You, and Darling (2014) view the life cycle as a set of stages the combination of which gives the idea of the engineering process Influencing life cycles. As it may be educed from their study, it is probable to enhance the asset performance and keep different processes influencing life cycles via different aspects. The authors’ understanding is close to what some other researchers suggest: a life cycle may be defined as the process of planning, creating, testing, and deploying a system, and each component can be influential (Hasan, Hossain, & Habib 2015). The costs associated with a certain life cycle are called, obviously, “life cycle costs.”
About the product connected with this particular life cycle, it is necessary to point out that it becomes the most important aspect in the context of the system based on PV technologies and promoted by many authors as the technology of the future addressing climate change issues and carbon dioxide emissions. In this context, as the researchers emphasize, they aimed at understanding the advantages and disadvantages associated with these technological innovations and used life cycle assessment to achieve their goal. Consequently, life cycle costs are considered by the aim.
As it was discovered, the production of PV modules consists of several stages.
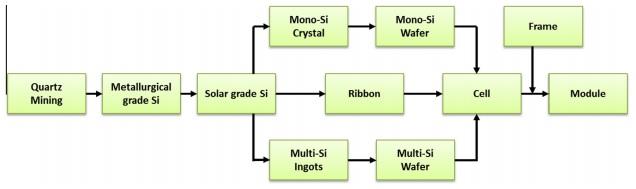
Flow diagram from raw acquisition to manufacturing stages of Si-PV modules (Yue, You, and Darling 2014, p. 671).
One can see that the authors follow the principle that the relationships between the life cycle cost drivers and the life cycle stages can be described in terms of the time framework. The processes that occur over time are represented by the line. As a rule, the life cycle contains two phases, acquisition and utilization, and several stages, such as conceptual design, detail design and development, production, and so on (Love et al. 2013). In this diagram, the researchers concentrate on the first stage and provide the details of how a PV module is being made.
In this context, it is necessary to refer to the term “cost drivers.” The life cycle cost drivers are recognized as the reason for the cost increase in the life cycle (Vikhorev, Greenough, & Brown 2013). In the process of the analysis, it is important to realize that it is the cause of the expenditure that should be examined, not the spending itself. Identifying the cause, one needs to make the search more effective by addressing the spheres of the most influence on overall costs (El-Akruti & Dwight 2013). Cost drivers are used in activity-based cost analysis since organizations, naturally, want to discover the causes before the beginning of their activity.
Taking into account the importance of cost drivers, it is probable to examine the stages associated with the acquisition phase and single out cost drivers, such as the costs of quartz mining, preparing metallurgical grade silicon, manufacturing solar grade silicon, etc. The analysis of the cost drivers is reasonable in the earliest stages since goal setting, assessment of resources and other significant procedures refer to the beginning of the life cycle. Thus, this approach seems to be relevant.
Further, the authors of the article address the energy payback time and energy return on energy investment. They calculate numerous indexes and argue that the electricity return from PVs depends on the domestic manufacturing scenario: in Europe and China, electricity mix (i.e. the proportion of different types of sources of energy) and energy efficiency differ since China relies on coal to a larger extent in comparison with the European countries and the USA that use renewable energy resources, such as hydropower plants, more (Yue, You, and Darling 2014). Consequently, costs are influenced. Europe and America tend to obtain better results. These data demonstrate that PV technology usage as one of the first-stage components becomes the most important factor that makes an impact on the total costs.
One more asset issue related to the life cycle costs under examination is connected with taxes. The researchers describe the break-even carbon tariff model for Si-PV modules based on the previous calculation as a preferable innovation (Yue, You, and Darling 2014). They suggest a formula that includes several parameters, and one of them, the local manufacturing cost, is influenced by the aspect that is the focus of this section, Si-PV modules as a product. Overall, this aspect is of primary importance to the life cycle cost associated with an asset. It may be stated that the researchers chose the right direction and managed to demonstrate how this aspect works and affects the life cycle costs.
Recommendations
From the perspective of the life cycle aspect discussed above, one can provide several suggestions connected with the cost drivers in the life-cycle analysis for practical usage in assets administration. While the authors themselves provide some key directions which may bring positive results and help reduce the costs, the list of recommendations may be enlarged. It will be advantageous to follow some of the strategies introduced in other countries, especially those that prove to be effectively using PV modules and demonstrate the improvement in terms of the energy payback time and energy return on energy investment.
- It is significant to draw attention to technology enhancement. As the researchers point out, the key characteristics of the Si-PV technologies, such as conversion efficiency, wafer thickness, and material utilization, should be amended (Yue, You, & Darling 2014). To bring technologies to perfection, one may combine the key parameters of the present-day technologies and encourage research in this sphere.
- Following the idea concerning the necessity to carry out research, it is vital to develop the policy that will adequately address the modern demand. The example of countries that developed such programs proves that they generate value: for instance, the USA launched a wide range of programs (PVUSA, Million Solar Roofs program, and so on) (Gambhir, Gross, & Green 2014).
- The third recommendation pertains to the later stages of PV module usage. As has been discussed above, China is notable for the high level of coal utilization, and this fact partially explains why the PV module’s efficiency is different in Europe and America and, on the other hand, China. Consequently, one of the urgent tasks is to handle the country’s electricity mix and implement technologies based on renewable energy resources.
Thus, taking into account the information above, one can state that life cycle cost drivers directly influence only the first stages when decisions and actions are planned. Ideally, they are projected taking into consideration possible changes connected with the external conditions that may either require changes or meet the expectations in detail. It can be said that the influence on other stages is indirect. Consequently, the hypothesis formulated at the beginning of the paper is not proven judging by the analysis of the large body of academic literature that exists nowadays and one of the articles. In this context, future research concerning the impact of life cycle cost drivers seems necessary.
Reference List
Blanchard, BC & Fabrycky, WJ 2011, Systems engineering and analysis, Prentice Hall, Upper Saddle River.
El-Akruti, K, & Dwight, R 2013, ‘A framework for the engineering asset management system’, Journal of Quality in Maintenance Engineering, vol.19, no. 4, pp. 398-412.
Gambhir, A, Gross, R, & Green, K 2014, The impact of policy on technology innovation and cost reduction: a case study on crystalline silicon solar PV modules, Web.
Hasan, MM, Hossain, M, & Habib, A 2015, ‘Corporate life cycle and cost of equity capital’, Journal of Contemporary Accounting & Economics, vol. 11, no. 1, pp. 46-60.
He, Z, & Xiong, W 2013, ‘Delegated asset management, investment mandates, and capital immobility’, Journal of Financial Economics, vol. 10, no. 2, pp. 239-258.
Karniouchina, EV, Carson, SJ, Short, JC, & Ketchen, DJ 2013, ‘Extending the firm vs. industry debate: does industry life cycle stage matter?’, Strategic Management Journal, vol. 34, no. 8, pp. 1010-1018.
Love, PE, Simpson, I, Hill, A, & Standing, C 2013, ‘From justification to evaluation: building information modeling for asset owners’, Automation in Construction, vol. 35, no. 1, pp. 208-216.
Ristimäki, M, Säynäjoki, A, Heinonen, J, & Junnila, S 2013, ‘Combining life cycle costing and life cycle assessment for an analysis of a new residential district energy system design’, Energy, vol. 63, no. 1, pp. 168-179.
Vikhorev, K, Greenough, R, & Brown, N 2013, ‘An advanced energy management framework to promote energy awareness’, Journal of Cleaner Production, vol. 43, no. 4, pp. 103-112.
You, F, Tao, L, Graziano, DJ, & Snyder, SW 2012 ‘Optimal design of sustainable cellulosic biofuel supply chains: multiobjective optimization coupled with life cycle assessment and input–output analysis’, AIChE Journal, vol. 58, no. 4, pp. 1157-1180.
Zakeri, B & Syri, S 2015, ‘Electrical energy storage systems: a comparative life cycle cost analysis’, Renewable and Sustainable Energy Reviews, vol. 42, no. 2, pp. 569-596.